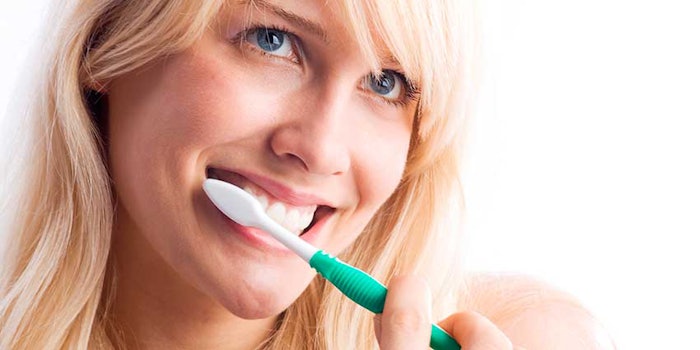
Pivotal research from the late 1950s continues to impact oral care technology development today. At that time, researchers at Indiana University successfully demonstrated the ability of fluoride to be incorporated into toothpaste and deliver an anticavity, or anticaries, benefit. The first toothpaste proven to be clinically effective against caries was the original Cresta brand toothpaste, a product formulated with stannous fluoride (SnF2) in an abrasive system that was only partially compatible with the fluoride active. Earlier toothpastes had combined ionic sodium fluoride, a highly reactive species, with calcium-based abrasives such as calcium carbonate. The active anticaries agent, the F- ion, reacted with free calcium in these formulations, forming insoluble calcium flouride, which produced clinically ineffective products.1, 2