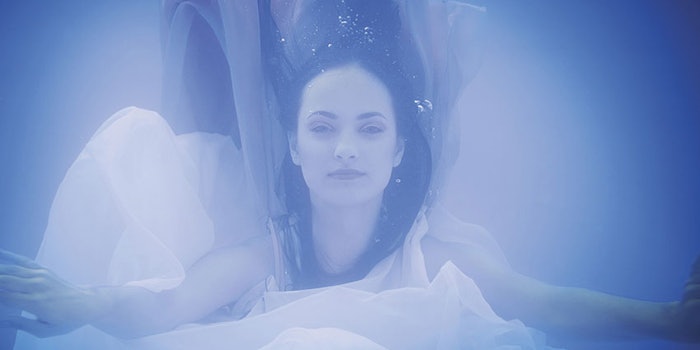
Editor’s note: the specifics of the ingredients and device examined herein are proprietary.
Log in to view the full article
Editor’s note: the specifics of the ingredients and device examined herein are proprietary.
*The work presented here was conducted during the author's time at TRI-Princeton; his affiliation has since changed.
The use of skin care products by consumers is ever-growing and evolving, and many actives and components that benefit the skin are incorporated to provide consumer-perceivable effects. The deposition, delivery and penetration of actives are key to the success of these products and consequential to their activity and efficacy.
Indeed, in many cases, delivery enhancements must be employed to assure the actives and benefit components reach deep into the layers of the stratum corneum to afford perceivably improved performance. Therefore, understanding and visualizing these parameters is essential both during product development and prior to final product commercialization, to provide scientific data in support of a product’s efficacy.
This paper explores attenuated total reflection Fourier transform infrared spectroscopy (ATR-FTIR) imaging methods, which are invaluable for analyzing the deposition and penetration of actives into skin layers. Here, skin care formulas containing actives were applied by two mechanical delivery systems: a device and manual application.
Subsequently, the surface and specific layers of skin were analyzed, in a semi-quantitative mode, to measure the effects of these approaches on the delivery of actives. In addition, molecular and structural areas of skin were investigated for levels of hydration in the different layers, to elucidate barrier function and the results by which these delivery mechanisms could change to improve the penetration of certain actives.
Experimental Protocol
Skin samples: For the described tests, ex vivo skin samples were prepared by two protocols. First, sequential tape-stripping produced fresh skin, which was surface analyzed by ATR; this indicates the penetration of actives into the stratum corneum. Second, the skin was microtomed into 2-6 µm thick skin sections and mounted on infrared (IR) windows in order to detect the penetration of actives into the respective epidermis, dermis or hypodermis layers.
Product application and ATR-FTIR: As noted, prior to analysis, formulas containing ingredients of interest were applied manually or using a mechanical device onto ex vivo porcine skin samples. Sequential tape-stripping was then employed and analyzed by ATR-FTIR imaginga.
The FTIR images were recorded with a spatial resolution of 1.5 µm and a spectral resolution of 4 cm-1. Two IR images were generated; one to determine relative levels of the active of interest and the other to visualize the hydration levels inside the stratum corneum. As noted, this served as an indicator of the active’s effects on skin barrier properties.
Application methods: Two application methods were analyzed for their impact on deposition and penetration, and compared to a control without treatment. Both application methods employed a pre-treatment cleansing stage to deliver identical formulations containing the same active ingredient of interest.
Method 1 used a mechanical device to assist the penetration of the active into skin. Method 2 merely employed manual application; both methods used the exact same formulation and active for investigation.
Use of a mechanical application device had a profound effect on the penetration of actives into successive skin layers.
ATR-FTIR Imaging
ATR-FTIR imaging uses a microscopic array across the skin surface, where each “pixel” is a distinct and individual FTIR spectroscopic scan capturing an IR fingerprint. By analyzing the skin surface after each tape strip, an FTIR spectroscopic “map” of the inside of the stratum corneum can be created. This map can be further analyzed for specific functional groups as absorbance peaks in the spectra, each of which is attributed to specific molecular species, structural entities or formulation components. These component spectral peaks are normalized against a consistent peak in the spectrum—in this case, the Amide I peak at approximately 1,645 nm, which is attributable to proteins found in the skin.
In Figure 1, the hyperspectral FTIR images of the skin’s cross-section, captured in transmission mode using microtomed skin layers on IR windows, clearly show the protein and lipid localization inside the skin. The skin surface view, captured using FTIR in reflection mode after sequential tape strips, shows a much lower resolution;1.55 µm per pixel compared with 6.25 µm per pixel for the transmission mode.
Delivery and Deposition of Actives
Figure 2 shows the hyperspectral images of various skin layers before and after treatment using two distinctly different delivery methods. Also shown is the control, for comparison. The peak ratio analysis is specific for the active of interest; for the control, it was water. As stated, Method 1 used a mechanical device for product application whilst Method 2 used manual application. In Figure 2, the peak area ratio of the active to Amide I is highest where it is red and lowest where it is blue; consequently, the control has no active and appears blue across all images.
As expected, prior to treatment and after cleansing, the active of interest was not present in skin samples. Also, since both methods of application used formulas containing the active, after immediate treatment (upon application) to the skin, the formulations were expected to show the active deposited onto the surface; the results concurred.
However, Method 1, using the mechanical device, showed greater deposition and penetration of the active down to layer 6 inside the stratum corneum. In contrast, Method 2, using manual application, showed deposition and penetration of the active, but did not achieve the same depth of penetration as Method 1. The untreated control did not show any deposition or penetration of active.
It should be noted that the levels of active penetration are not exclusive to Method 1, although Method 1 did impact the penetration of actives deeper into skin. Also, the levels of active penetrating via Method 2 seem to be just as evident in skin layers 1 and 2 as they are using Method 1; although with Method 1, penetration into layers 1 and 2 was much more pronounced and layers 4 and 6 show significant delivery of the active.
Therefore, the use of the mechanical device had a profound effect on the penetration of the active and enhanced its deposition and delivery into successive layers of the skin. It can then be concluded that this delivery system (Method 1) had an effect on the active of interest by improving its deposition and penetration into the skin.
Only in the case where moisturizer was applied by the mechanical device was a major increase in hydration at each skin layer depth observed.
Impact of Hydration on Actives Delivery
Prior to treatment, the surface of the stratum corneum showed lower water content levels, as evidenced by the lack of significant deeper green to red colors (see Figure 3). Examining the untreated controls, these lower relative hydration levels were true for all skin layers and depths studied. However, immediately after treatment using either the control moisturizer or test moisturizers applied by the two methods, only Method 1 using the mechanical device showed a major increase in the hydration at each skin layer depth. This is evidenced by the hyperspectral imaging into the deeper green and red colors for the majority of layers.
This increased water content was associated with the delivery of the active of interest by Method 1 into layer 6 of the skin. The water content also seemed to increase slightly with Method 2; especially at the surface and into the first two layers of skin. Again, this increase in hydration is associated with the layers where the active of interest was found. These results seem to indicate that the mode of action for the skin care product device is to increase the deposition and delivery of the active into the skin, which thereby allows the active formulation to increase hydration in the layers of the stratum corneum where penetration occurs.
This increase in water content and hydration of the deeper layers of the skin may be an indicator of a more pronounced barrier function, which could be due to the active formulation’s influence and efficacy. Therefore, the increased delivery and penetration efficacy of Method 1 may lead to a more robust barrier function at deeper levels than is expected when the active of interest remains on the surface (as in Method 2).
Conclusions
Here, a mechanical skin care application device was shown to enhance the deposition and delivery of an active into successive layers of the skin. It also was concluded that the active of interest had effects on the water levels (hydration) in layers of the skin, and that this may be indicative of the efficacy of the active formulation. Therefore, improvements in the deposition and delivery of the test active into the skin could be expected to improve hydration and thereby barrier function in the layers where the active formulation is delivered.
Finally, the ATR-FTIR imaging methodology used in this study was demonstrated as a useful means to assess the delivery, deposition and penetration of benefit agents into the skin, to determine the relative efficacy and mechanisms of systems that are designed to enhance this delivery.
Acknowledgements: This study was conducted at TRI-Princeton Laboratories, whose authors wish to thank Surbhi Mittal for expert technical assistance.