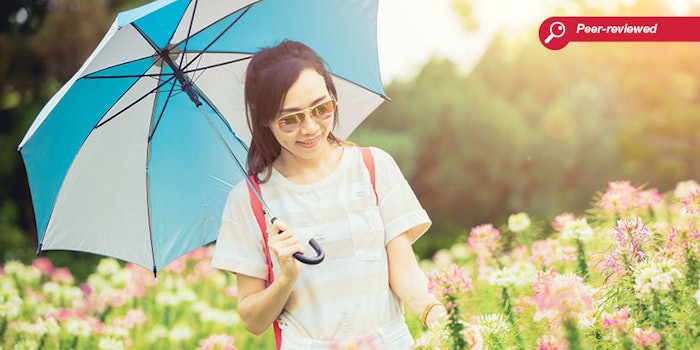
Read this article in its entirety in the October 2020 digital edition. . .
The cumulative body of research today establishes that skin aging is driven by exposure to key environmental stressors such as solar light, air pollution, tobacco smoke and cosmetics, which mediate damage largely through the formation of excess ROS.1-4 This advocates for a strategy that topical formulas designed to care proactively for skin on a daily basis, including moisturizers, therapeutic products or sunscreens, should incorporate ingredients as prophylactics to reinforce skin’s ability to cope with excess ROS formation. This is especially true considering that:
• Exposure to sun or air pollution generates such an abundance of ROS in skin that its intrinsic antioxidant defenses are overwhelmed, which then allows an excess of ROS to build up within skin.5-7
• Even high SPF broad-spectrum sunscreens cannot prevent sun-induced ROS formation within skin—especially considering at least 50% of sun-induced ROS originate from spectral regions (visible or IR) outside of the protective capacity of sunscreens.8, 9
• Of all environmental stressors, sun exposure is the most potent generator of ROS in skin, accounting for an estimated 80% of the visible signs of skin aging.10
• Exposure to air pollution in the form of particulate matter correlates significantly with hyperpigmentation and wrinkle formation.11
• Sun exposure induces an immediate release of iron ions within skin via the Fenton reaction that are believed to be a major source of ROS.12
• ROS have been hypothesized as critical precursors in novel pathways of DNA dimer formation that occurs in skin even hours after sun exposure ends. This has new implications for skin cancer development.13-15
Left unchecked, excess ROS can oxidize cellular targets such as lipids, proteins, DNA and mitochondria; affect cellular signaling; and influence gene expression pathways, all of which create oxidative stress, perturb barrier function and stimulate increases in matrix metalloproteinases (MMPs) and pro-inflammatory cytokines.16-23 Activation of MMPs leads to the degradation of collagen, skin’s major structural protein, and also inhibits new collagen synthesis. Persistent, chronic induction of inflammatory cytokines leads to a state of ongoing low-grade (i.e., sub-clinical) inflammation, or inflammaging, another recognized contributor to aging.24
The good news is ROS levels created within skin from exposure to environmental stressors can be managed effectively. As shown in Figure 1, external stressors typically induce ROS formation through interaction with endogenous molecules within skin. These form intermediates that then give rise to ROS. The attenuation of excess ROS formation can therefore be accomplished by using antioxidants to neutralize ROS once they are formed but also before they can react to cause damage—by using physical quenchers that interact with the intermediates in a way that restores the endogenous molecules to their original state.
Antioxidants are further challenged by the diversity of ROS with different chemical structures and reactivities, which include both free radicals and non-radical molecules (see Table 1). Conventional antioxidants typically show high reactivity toward free radicals with unpaired electrons (•OH, •OOR), which they neutralize via the donation of an H atom (H•) to restore their original chemical stability. However, other highly reactive non-radical ROS (1O2, ONOO-) confer new structural requirements on antioxidants to endow them with broader and more effective capabilities.
In this article, combinations of in vitro chemical reactions and cellular assays under stress from UV, blue light or urban dust particulate matter are used to assess the multiple ways by which a single ingredient, acetyl zingerone [3-(4-hydroxy-3-methoxybenzyl)-pentane-2,4-dione], with its unique molecular design, can help to manage excess ROS levels.
Materials and Methods
Acetyl zingerone: The synthesis and identification of acetyl zingerone (AZ) has been described elsewhere.25 The producta, used in the present study, was > 99% pure. In addition, a-tocopherolb (96.5% pure) and trans-resveratrolc (100% pure) were procured for comparison.
ROS quenching: The in vitro methods used to measure the performance of AZ to neutralize ROS or physically quench singlet oxygen were reported previously.25 Additional tests comprised the following.
Photostability assessment: AZ, a-TOC and resveratrol were solubilized in ethanol or aqueous ethanol and aliquots were irradiated with 13 J cm-2 of UVB and UVA radiation using a photochemical reactord to simulate daylight conditions. Extents of degradation were monitored by an HPLC method described25 elsewhere.
Fluorometric iron chelation assay: A fluorometric assay modeled on the method reported by Ou, et al., was employed.26 This assay uses fluorescein as a sensitive probe for hydroxyl radical, which reacts rapidly with fluorescein to destroy its fluorescent properties. Loss of fluorescence over time was then used to monitor the effectiveness of different chelators to inhibit the formation of hydroxyl radical as FeCl2 (200 μM) reacted with H2O2 (0.055 μM) via the Fenton reaction in PBS at pH 7 by chelating and removing Fe+2 as a reactant. Chelating agents were dissolved in ethanol and diluted to concentration in PBS buffer at pH 7 to yield assay concentrations of 600 μM for AZ, AZ methyl ether and Deferiprone; or 200 μM for EDTA, EDDS or Desferal.
Physical quenching of AGEs: The ability of AZ to physically quench photoexcited states of advanced glycation end products (AGEs) was performed by following the plasmid DNA cleavage assay reported by Wondrak et al.27 Briefly, 200 ng of fX-174 RF bacteriophage supercoiled DNA was irradiated in the presence of 25 ng of filtered glycated bovine serum albumin (20 mg/mL BSA reacted for 3 weeks with 180 mg/mL glucose at 37°C) in a final volume of 100 μL.
Irradiation was performed in a 96-well plate without the lid using a UVA lampe at 6.4 mW/cm2 for 2 hr. Following irradiation, 10 μL of the sample was mixed with 2 μL of loading buffer, resolved in 1% agarose gel (at 90 V for 20 min) and stained with ethidium bromide. Bands from supercoiled and uncoiled DNA were visualized using a transilluminatorf and photographs of the gels were processedg to calculate a ratio of unwound to supercoiled DNA.
Intracellular ROS in keratinocytes: Measurement of intracellular ROS was carried out in cell cultures of human epidermal keratinocytes grown using a mediumh (60 uM calcium) supplemented with 0.2% bovine pituitary extract, 1 μg/mL recombinant human insulin-like growth factor-1, 0.18 μg/mL hydrocortisone, 5 μg/mL bovine transferrin and 0.2 ng/mL human epidermal growth factor, which were incubated at 37 ± 2°C and 5 ± 1% CO2. After sufficient cell numbers were grown, they were seeded into 96- or 24-well plates and grown to confluency, loaded with 2’, 7’-dichloro-dihydrofluorescein diacetate (DCF-DA), then rinsed with PBS.
Blue light exposure: Cells were treated with either AZ at different concentrations prepared in a media of phenol red-free Hanks Balanced Salt Solution, or the media alone as an untreated control. They were then irradiated with blue light at a distance of 25 cm in a humidified incubator at 37± 2°C. The blue light source comprised a panel of 225 blue LED lights arranged in a 15 cm x 15 cm square with an output of 22 watts of 450 nm blue light.
Baseline measurements were recorded on the underside of the wells prior to irradiation with a fluorometerj having an excitation wavelength of 485 nm and an emission wavelength of 518 nm. Cells were irradiated for 30 min and 60 min, which corresponds to ~1 hr to 2 hr of outside midsummer sunlight, based on solar irradiance in the visible range9 of about 50 mW cm-2, and the fluorescence was recorded. Non-irradiated cells were also placed in the incubator but were covered with aluminum foil to block blue light exposure.
Urban dust exposure: Cells were treated with 10 μL of a 10 mg/mL stock solution of urban dustk prepared in supplementedg media or media alone as the untreated control. Baseline fluorescence measurements were recorded as described previously. Cells were incubated for 3 hr with fluorescence measurements recorded at 1 hr, 2 hr and 3 hr time points.
Lipid peroxidation: To test effects against urban dust-induced lipid peroxidation, cells were treated with urban dust as before, then placed in the incubator for 4 hr. After 3.5 hr, cells were treated with 10 μL of 100 μM peroxidation sensorm and incubated for an additional 30 min. This sensor localizes in cell membranes and upon reaction with lipid hydroperoxides, becomes highly fluorescent. One set of cells was not treated as a blank to correct for background fluorescence. At the end of the incubation period, cells were washed three times with PBS then read with a fluorometer using 485 nm excitation and 518 nm emission wavelengths.
Results: Neutralizing Radicals
Inspired by curcumin and zingerone from ginger, the molecular structure of AZ is unique (see Figure 2), which bestows the molecule with unique capabilities to attenuate different forms of ROS with high efficiency (see Table 2). Compared with a-tocopherol (a-TOC) and resveratrol, two antioxidants commonly used in topical products, the effectiveness ratios show AZ was superior by factors ranging from 2.7 to 126 against a-TOC, and by 157 to 469 against resveratrol.
The ability of AZ to neutralize peroxynitrite and singlet oxygen, two of the most reactive forms of ROS, with high efficiencies is noteworthy. Research establishes that peroxynitrite can react both as an oxidant or a nucleophile28 and as such, undergoes facile reaction with enolizable carbonyls such as 3-methyl-2,4-pentanedione.29 Inclusion of this functional group on AZ equips it with exceptional capabilities, compared with a-TOC or resveratrol, to scavenge ONOO- with high efficiency.
Similarly, the chemical structure of AZ also promotes the neutralization of 1O2 through physical quenching, as opposed to a chemical reaction. Since 1O2 is an electronically excited state species of molecular oxygen (3O2), it can be deactivated either through physical quenching or chemical reaction. Physical quenching restores 1O2 back to its ground state (3O2) without being consumed in the process. In contrast, deactivators that work through chemical reactions become sacrificed.
Many organic compounds that neutralize 1O2 have been shown to work through a combination of physical quenching and chemical reaction; however, physical quenching appears more favorable for electron-rich aromatics containing phenol or methoxy groups, both of which reside on AZ.30, 31 The mechanism of AZ and resveratrol to function mainly as physical quenchers is illustrated by the data in Table 3, which shows that during the period of 1O2 exposure, a-TOC undergoes significantly more chemical degradation than either AZ or resveratrol. Deactivation of 1O2 through physical quenching is a key advantage of AZ over traditional antioxidants.
Results: Selective Chelation
In addition to effectively neutralizing •OH, AZ also impedes •OH production by virtue of its ability to chelate iron as a participating reactant in the Fenton reaction (see Equation 1):
Fe+2 + H2O2 → Fe+3 + •OH + _OH Eq. 1
Exposure of skin to UVA causes an immediate release of iron from the iron storage protein ferritin,12 which then reacts with hydrogen peroxide to form •OH. This is postulated as a major source of •OH within the skin caused by sun exposure and consequently, a major contributor to photo-oxidative stress.32
The potential of AZ to inhibit the Fenton reaction was monitored in simple reactions of Fe+2 with H2O2 by monitoring the loss in fluorescence of fluorescein. One might argue that the loss of fluorescence results from AZ’s chelation as well as its •OH-neutralizing abilities. In order to dispel this ambiguity, similar experiments were carried out with AZ methyl ether, which prevents •OH neutralization.
. . .Read more in the October 2020 digital edition. . .
References
- Krutmann, J., Bouloc, A., Sore, G., Bernard, B.A. and Passeron, T. (2017). The skin aging exposome. J Dermatol Sci 85 152-161.
- Parrado, C., Mercado-Saenz, S., Perez-Davo, A., Gilaberte, Y., Gonzalez, S. and Juarranz, A. (2019). Environmental stressors on skin aging. Mechanistic insights. Front Pharmacol 10 759; doi: 10.3389/fphar.2019.00759
- Polefka, T.G. and Meyer,T.A. (2017). Cutaneous oxidative stress and aging, in: Farage, M., Miller, K. and Maibach, H., eds, Textbook of Aging Skin. Springer, Berlin, Heidelberg 651-676.
- Dupont, E., Gomez, J. and Bilodeau, D. (2013). Beyond UV radiation: A skin under challenge. Int J Cosmet Sci 35 1-9.
- Jin, S.P., Zhenyu, L., ... Cho, S., et al. (2018). Urban particulate matter in air pollution penetrates into the barrier-disrupted skin and produces ROS-dependent cutaneous inflammatory response in vivo. J Dermatol Sci 91 175-183.
- Rhie, G., Shin, M.H., ... Chung, H.J.H., et al. (2001). Aging and photoaging-dependent changes of enzymic and nonenzymic antioxidants in the epidermis and dermis of human skin in vivo. J Invest Dermatol 117 1212-1217.
- Thiele, J.J., Traber, M.G. and Packer, L. (1998). Depletion of human stratum corneum vitamin E: An early and sensitive in vivo marker of UV induced photo-oxidation. J Invest Dermatol 110 756-791.
- Zastrow, F.L., Groth, N., Klein, F., Kockott, D., Lademann, J., Renneberg, R. and Ferraro, L. (2009). The missing link – light induced (280 nm–1600 nm) free radical formation in human skin. Skin Pharmacol Physiol 22 31-44.
- Liebel, F., Kaur, S., Ruvolo, E., Kollias, N. and Southall, M.D. (2012). Irradiation of skin with visible light induces reactive oxygen species and matrix-degrading enzymes. J Invest Dermatol 132 1901-1907.
- Flament, F., Bazin, R., Laquieze, S., Rubert, V., Simonpietri, E. and Piot, B. (2013). Effect of the sun visible clinical signs of aging in Caucasian skin. Clin Cosmet and Invest Dermatol 6 221-232.
- Vierkotter, A., Schikowski, T., Rannft, U., Sugiri, D.,Matsui, M., Kramer, U. and Krutmann, J. (2010). Airborne particle exposure and extrinsic skin aging. J Invest Dermatol 130 (12) 2719-2726.
- Pourzand, C., Watkin, R.D., Brown, J.D. and Tyrrell, R.M. (1999). Ultraviolet A radiation induces immediate release of iron in human primary skin fibroblasts: The role of ferritin. Proc Natl Acad Sci USA 96 6751-6756.
- Premi, S., Wallisch, S., ... Brash, D.E., et al. (2015). Chemiexcitation of melanin derivatives induces DNA photoproducts long after UV exposure. Science 347 842-847.
- Delinasios, G.J., Karbaschi, M., Cooke, M.S. and Young, A.R. (2018). Vitamin E inhibits the UVAI induction of “light” and “dark” cyclobutane dimers and oxidatively generated DNA damage in keratinoctyes. Scientific Reports 8, 423; doi:10.1038/s41598-017-18924-4
- Lawrence, K.P., Douki T., Sarkany, R.P.E., Acker, S., Herzog, B. and Young, A.R. (2018). The UV/Visible radiation boundary region (385-405 nm) damages skin cells and induces “dark” cyclobutane pyrimidine dimers in human skin in vivo. Scientific Reports 8, 423; doi: 10.1038/s41598-018-30738-6
- Xu, F. and Fisher, G.J. (2002). Ultraviolet (UV) light irradiation induced signal transduction in skin photoaging. Arch Dermatol 138 1462 -1470.
- Kimball, A.B., Alora-Palli, M.B., ...Tanura, M., et al (2018). Age-induced and photoinduced changes in gene expression profiles in facial skin of Caucasian females across 6 decades of age. J Am Acad Dermatol 78 (1) 29-39.
- Tu, Y. and Quan, T. (2016). Oxidative stress and human skin connective tissue aging. Cosmetics 3, 28 1-12.
- Rinnerthaler, M., Bischof, J., Strueubel, M.K., Trost, A., and Richter, K. (2015). Oxidative stress in aging human skin. Biomolecules 5 545-589.
- Stout, R. and Birch-Machin, M. (2019). Mitochondria’s role in skin aging. Biology 8, 29; doi: 10.3390/biology8020029
- Dong, K.K., Damaghi, N., ... Yarosh, D.B., et al. (2008). UV-induced DNA damage initiates release of MMP-1 in human skin. Exp Dermatol 17 1037-1044.
- Chiba, K., Kawakami, K., Sone, T. and Onoue, M. (2003). Characteristics of skin wrinkling and dermal changes induced by repeated application of squalene monohydroperoxide to hairless mouse skin. Skin Pharmacol Appl Skin Physiol 16 242-251.
- Biniek, K., Levi, K. and Dauskardt, R.H. (2012). Solar UV radiation reduces the barrier function of human skin. Proc Natl Acad Sci 109 1-6.
- Zhuang, Y. and Lyga, J. (2014). Inflammaging in skin and other tissues—The roles of complement system and macrophage. Inflamm Allergy Drug Targets 13 153-161.
- Chaudhuri, R.K., Meyer, T., Premi, S. and Brash, D. (2020). Acetyl zingerone: An efficacious multifunctional ingredient for continued protection against ongoing DNA damage in melanocytes after sun exposure ends. Int J Cosmet Sci 42 (1) 36-45.
- Ou, B., Hampsch-Woodill, M., Flanagan, J., Deemer, E.K., Prior, R.L. and Huang, D. (2002). Novel fluormetric assay for hydroxyl radical prevention capacity using fluorescein as the probe. J Agric Food Chem 50 2772-2777.
- Wondrak, G.T., Jacobson, M.K. and Jacobson, E.L. (2005). Identification of quenchers of photoexcited states as novel agents for skin photoprotection. J Pharmacol Exp Ther 312 482-491.
- Radi, R. (2013). Peroxynitrite, a stealthy biological oxidant. J Biol Chem 288 26464-26472.
- Knudsen, F.S., Pernatti, C.A.A., ... Bechara, E.J.H., et al. (2000). Chemiluminescent aldehyde and b-diketone reactions promoted by peroxynitrite. Chem Res Toxicol 13 317-326.
- Darmanyan, A.P. and Jencks, W.S. (1998). Charge-transfer quenching of singlet oxygen O2 (Δg) by amines and aromatic hydrocarbons. J Phys Chem A 102 7420-7426.
- Mukai, K., Daifuku, K., Okabe, K. Tanigaki, T. and Inoue, K. (1991). Structure-activity relationship in the quenching reaction of singlet oxygen by tocopherol (vitamin E) derivatives and related phenols. Finding linear correlation between the rates of quenching of singlet oxygen and scavenging of peroxyl and phenoxyl radicals in solution. J Org Chem 56 4188-4192.
- Thomas, C., Mackey, M.M., Diaz, A.A. and Cox, D.P. (2013). Hydroxyl radical is produced via the Fenton reaction in submitochondrial particles under oxidative stress: Implications for diseases associated with iron accumulation. Redox Report 14(3) 102-108.
a Synoxyl AZ (INCI: Acetyl Zingerone), Sytheon
b Millipore Sigma
c Nutrivita
d Raynonet RPR-100, Southern New England Ultraviolet Company
f Hoefer
g NIH ImageJ
h EpiLife
j Fluoroskan Ascent
k Sigma Chemicals, standard reference material 1649b
m Image-It Lipid Peroxidation Sensor