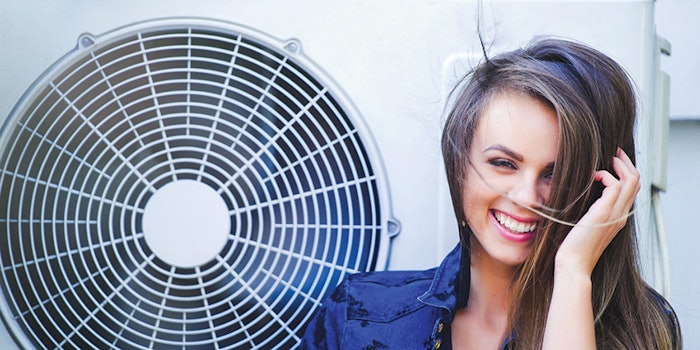
*Republished with permission from the Journal of Japan Hair Science Association (in Japanese)
Silicones have a long history of use in beauty and personal care products, extending back to the early 1950s. These versatile polymers, which comprise silicon and oxygen atoms, represent a broad chemical family. They can form structures with almost endless variations and a range of useful properties. For example, modifications can be made by altering their molecular weight or by adding organic functionalities, such as polyether, alkyl and amine. The resulting materials include volatile fluids, gums, waxes and elastomers, and they can be oil or water soluble.
Silicones offer several useful characteristics for both consumers and formulators, including:
- Ease of spreading, with a smooth, silky and lasting afterfeel on skin and hair;
- Water repellency;
- Hair shine, smoothness and improved combing;
- A broad range of colorless, transparent and odorless forms;
- Emulsion options for ease of formulation; and
- An extensive history of testing and safe use.
Shampoos were first formulated with silicones more than 30 years ago. With advancing silicone technology, these ingredients came into broader use for hair cuticle protection and conditioning, similar to earlier-used mineral and vegetable oils or fatty acid esters.
When hair becomes damaged, its fiber surfaces become hydrophilic.1 This leads to roughness and a feeling of “drag” and stiffness during combing, along with tangling.2 This hydrophilization of the hair surface is due to a loss of the F-Layer, which mainly comprises the fatty acid 18-methyleicosanoic acid (18-MEA). Chemical treatments such as bleaching or perming may remove this component of the F-layer.
It has been reported3-5 that in a single permanent waving treatment, approximately 50% of the 18-MEA content is lost; in a bleaching treatment, almost all of it is lost. Further, in temporary coloring products based on acid processes, 18-MEA is removed due to ingredients such as benzyl alcohol, which acts as a carrier for colorant into the fiber. Exposure to UV also has been reported to cause the removal of 18-MEA.5
To help reduce such hair damage, products with conditioning benefits were developed. Silicone and cationic cellulose are among the main materials formulated for this purpose, and when diluted with water, these ingredients form a positively charged coacervate that deposits on the hair surface.
Beginning in 1992, nonscientific reports began to circulate suggesting silicones in two-in-one shampoos may negatively impact the effect of oxidative hair dyes and permanent waves, as well as block pores. These reports became more visible via the use of the web—and later with social media and blogs—leading to silicone-free claims in specific variants of commercial products. These inaccuracies likely reflect a misunderstanding of the mechanism of action of silicones.
As such, the current study explores changes in hair texture due to silicone-containing shampoo formulations, as well as their effects on the scalp. Moreover, the impact of silicone-containing shampoo formulations on both oxidative hair dyeing by acidic dyes and permanent wave formulations is described.
Understanding Coacervates
Cationic cellulose is obtained by imparting a cationic character to cellulose, a polysaccharide (see Figure 1). Cellulose itself is derived from plants and modified to become soluble in water. Cationic cellulose is obtained by transforming a percentage of the alcoholic sites on the polymer into cationic sites via alkylation with, for example, glycidyltrimethylammonium chloride. The impact on the performance, i.e., soft feel on the hair when rinsing, varies according to the degree of cationic character (α) of the cellulose or anionic charge of the surfactant.6-14
The cationic polymer present in shampoo formulations forms an ion complex with anionic surfactants. The morphology of the ion complex varies significantly with the surfactant concentration and degree of micelle concentration (see Figure 2).7, 8 At an anionic surfactant concentration below its critical micelle concentration (CMC), the cationic polymer forms a transparent solution, since few surfactant molecules are in the bonded state with the cationic polymer (see Figure 2, Stage 1). The ion complex separates in the region where the cationic charge of the cationic polymer is neutralized by the anionic surfactant (see Figure 2, Stage 2). This phenomenon of separation of an ion complex is called coacervation, and the separated product is called a coacervate. Moreover, at high concentrations of anionic surfactant, many micelles are bonded to the cationic polymer and the solution becomes transparent again (see Figure 2, Stage 3).
In contrast, when typically high starting concentrations of anionic surfactant—such as in a shampoo (Stage 3)—are reduced by dilution, the ion complex dissolution state shifts toward Stage 2.11-14 At this level, the structure of the ion complex changes according to the balance between the cationic charge of the cationic polymer and the anionic charge of the anionic surfactant.11 The structure of the ionic complex is determined by the charge of the cationic polymer and the anionic surfactant/amphoteric surfactant micelle, and the feel of the shampoo changes accordingly. The higher the charge of the cationic polymer and the anionic surfactant/amphoteric surfactant micelle, the more intricate and closely packed the structure, and the thicker the film. When both charges are weak, however, an ion complex having a loose structure and containing gaps is formed. The feel and thickness also changes accordingly.
To reduce hair damage, conditioning products including silicone and cationic cellulose were developed, which form a positively charged coacervate that deposits on the hair.
If the shampoo is used when the hair is adequately wet, it is diluted seven- to tenfold and is further diluted upon rinsing.15 At this stage, the number of bonded micelles decreases, and a crosslinked structure in which the micelle engulfed in the cationic polymer forms. When the cationic charge of the polymer and the anionic charge of the surfactant are both high, the crossl-inking increases considerably to afford a closely-packed ion complex film. When both charges are low, the cross-linking decreases and an ion complex with a loose network structure forms. The thickness of the coating on the hair surface, or the feel at the time of rinsing, thus changes according to the structure of the ion complex. Once separated, the coacervate does not revert to Stage 1 by dilution upon rinsing. Also, when the shampoo is diluted abruptly to Stage 1 (100 times or more), the coacervate does not form and does not separate from the system.
Shampoo Dynamics
A shampoo formulation typically consists of 10-15% surfactants—not only anionic, but also amphoteric—plus, approximately 0.5% cationic polymer such as the cationic cellulose. Moreover, oil-based ingredients such as silicone or jojoba oil are dispersed in the shampoo for emollient effects to preserve moisture, softness and similar nurturing characteristics.
Shampoos also include nonionic surfactants such as foaming agents or amphoteric agents, which can help control the performance of the product. It is therefore possible to change the structure of the coacervate complex8 by controlling the anionic charge per micelle based on their proportion, and by controlling the cationic charge based on the cationic character of the cationic polymer.
During dilution, the shampoo forms a hydrophobic coacervate and is deposited on the hair via hydrophobic interactions with the hydrophobic hair surface, thus giving a better feel upon rinsing. On the other hand, the coacervate plays the role of a carrier for efficient deposition of other dispersed active ingredients. The dispersed ingredients are incorporated during coacervate formation and are deposited on the hair along with coacervate via hydrophobic interactions.8, 13, 14
Coacervate Formation and Deposition
Coacervate verification: Before assessing its deposition onto hair, coacervate formation was first verified using silicone as a marker (see Figure 2). Formulations containing 10% and 15% of an anionic surfactant and amphoteric surfactant (see Formula 1) were diluted seven- and tenfold, respectively. The amount of coacervate was measured based on the extent of turbidity using a spectrophotometer (see Figure 3), and in all cases, coacervate formation was confirmed; the maximum amount was formed with the 10% formulation diluted sevenfold and the minimum with 15% formulation diluted tenfold.
Hair treatment protocol: Next, to measure the deposition of coacervate onto the hair surface, healthy black hair of Japanese origin was first soaked for 1 min at room temperature (RT) in the 10% diluted test formula (see Formula 1) or its undiluted equivalent, for comparison, then rinsed with warm water at 40°C for 1 min. Hair was then towel-dried and air-dried.
Deposition via EDX: To measure the deposition of coacervate, energy dispersive x-ray spectroscopy (EDX) was performed on the treated hair. EDX is primarily used to obtain elemental information on an outermost surface. Interestingly, the observed quantity deposited from the 10% dilution was significantly more than from its undiluted equivalent (see Figure 4). The authors concluded this was due to coacervate formation, explained in https://www.cosmeticsandtoiletries.com/articleaddons/500171591.html and previously demonstrated.11 This indicated the amodimethicone present in the coacervate was deposited onto the hair surface.14
Deposition measured via TOF-SIMS: Similar experiments were conducted using dimethicone, but measurements via EDX could not detect its deposition. Since dimethicone is used more frequently in shampoo formulations than amodimethicone, measurements were pursued further using time-of-flight secondary ion mass spectrometry (TOF-SIMS), which has a higher sensitivity than EDX and an extremely small depth of analysis of about 1 nm.16
In TOF-SIMS, secondary ions generated from the outermost surface by a pulsed ion beam are analyzed by means of a time-of-flight mass spectrometer, for obtaining information about the chemical structure of the outermost surface. As shown in Table 1, TOF-SIMS revealed that approximately one layer of dimethicone was present, attributed once again to the carrier effect of coacervation.14
Hair Friction Test
Next, the coefficient of dynamic friction was assessed for hair washed and dried using the 15% surfactant-containing shampoo described in Formula 1, adapted according to A through F in Figure 5.14 Ten hair strands pasted on a glass slide at 1 mm intervals were used as the samples. Each sample was placed on a friction testera and the frictional force was measured while moving the contact element at 1 mm/s with a 25-g weight (n = 3). Measurements of frictional force were performed at 25°C and 50% RH.
Reduced friction was confirmed for all formulations containing cationic cellulose. After drying, however, the cationic cellulose generally did not impart a slippery or smooth feel on the hair, depending on the formulation. In contrast, treatment with a silicone-containing shampoo improved the slippery, smooth feel after drying (see Figure 5); similar results were obtained using the 10% shampoo (data not shown).
Oxidative Dye and Permanent Wave Protocols
Next, sample tresses of healthy black hair from Japanese subjects were treated with the 15% surfactant shampoo (see Formula 1), followed by an oxidative hair dye (see Formula 2) or permanent wave treatment (see Formula 3). Similar to before, hair was soaked in the test shampoo for 1 min at RT, rinsed with warm water at 40°C for 1 min, then towel-dried and air-dried.
Even after the deposition of dimethicone due to coacervation, hair dye color was not affected.
Oxidative dye treatment: The oxidative hair dye was obtained by mixing equal amounts of Solutions 1 and 2 in Formula 2, and then used to soak the preshampooed hair at 30°C for 30 min. Thereafter, hair was again washed but with a 15% shampoo formulation excluding silicone, rinsed with warm water at 40°C for 1 min, and towel-dried and air-dried again.
Permanent wave treatment: For the permanent wave process, preshampooed hair (as described previously) was soaked in Solution 1 of the permanent wave treatment at 30°C for 15 min. Thereafter, the treated hair was rinsed with warm water at 40°C for 1 min and toweled dry. Following, towel-dried hair was soaked in Solution 2 of the permanent wave treatment at 30°C for 15 min; following, it again was towel-dried and air-dried.
Results: Coacervate vs. Dye and Perm Formulas
Using a spectrophotometer, the authors found that even after the deposition of dimethicone due to coacervation, hair dye color was not affected; per L, a, b measurements with and without silicone (see Figure 6). Similarly, hair treated with the permanent-wave solution containing thioglycolic acid as the main ingredient (see Formula 3) was evaluated by the Kirby method.17 When dimethicone was deposited onto hair due to the carrier effect of the coacervate, the wave effect remained unchanged (see Figure 7). A similar result was obtained with cysteine as the main ingredient of the permanent wave.
Although not shown here, similar investigations were made for a combination of dimethicone and amodimethicone as well as petroleum jelly in place of dimethicone, but no significant differences in oxidative hair dyeing or permanent wave effects were noted.
Effects on the Scalp18
Finally, the potential for silicone to plug scalp pores was evaluated by measuring the permeation of lidocaine into pig skin, which was prepared by hair-plucking to expose the pores. Lidocaine, one of the most commonly used local anesthetics, is known for its high cutaneous permeability in molecular form. It exists in ionic form at pH 5.0, which is less than its isoelectric point (pI, 7.9), and, therefore, cannot penetrate the highly fat-soluble stratum corneum. Consequently, its main route for penetration is through pores of the skin. At a pH higher than the isoelectric point (pH 10.0), lidocaine is in molecular form, which has higher hydrophobic character compared to the ionic form, so it can penetrate the stratum corneum. Thus, by using the ionic form of lidocaine (pH 5.0), which follows skin pores as its main route of penetration, it could be determined whether or not various silicone emulsions and silicone-blended shampoos blocked skin pores.
A silicone emulsionb or the same 15% test shampoo formula (see Formula 1) was used to treat skin, and the penetration of the drug into skin was assessed.
Results demonstrated that even when the silicone emulsion was directly coated onto pig skin, the pores were not blocked when washed with warm water at 40°C. Further, when the skin was coated with foam from the 15% shampoo formulation for 1 min, then rinsed for 30 sec, the degree of permeation was similar to that of with a pure-water coating (see Figure 8). It was therefore concluded the silicone-blended shampoo did not block skin pores under normal use.
Conclusions
The mechanism underlying coacervate formation acts by means of an ion complex of an anionic surfactant and cationic cellulose. The resulting sensory effects due to silicone deposited on the hair surface have been outlined, including slippery and smooth sensory performance. Moreover, silicone does not appear to influence oxidative hair dyeing or permanent wave treatments. Additional results determined that silicone-blended shampoos do not block skin pores.
From the consumer perspective, the combined sensory aspects of hair are judged by combing or running fingers through the hair. For each individual, hair condition on the front and back sides of the head can be noticeably different. Moreover, for the same hair, its condition at the root, along the extended fiber, or tip, is different. Hair condition also differs with the cross section of a hair fiber, whether in the cuticle, cortex or medulla. Scientists tend to follow the path of analysis or research at the micro level, but where product formulations and development are concerned, macro-level evaluation is imperative. Thus, identifying the reasons for the sensory aspects of hair feel; verification by macro analysis; and translating these concepts to innovative products are vital.
Once lost, 18-MEA cannot be reproduced on the hair surface. Hence, hair care products such as shampoos play a major role in preserving this lost component of hair fiber. Scientific analysis of hair care processes, and the expanded knowledge obtained, will allow the development of next-generation hair care products for today’s wide range of global consumers.
References
- ML Tate, YK Kamath, SB Ruetsch and HD Weigmann, Quantification and prevention of hair damage, J Cosmet Sci 44(6) 347-371 (1993)
- H Tanamach, S Tokunaga, N Tanji, M Oguri and S Inoue, 18-MEA and hair appearance, J Cosmet Sci 61(2) 147-16 (2010)
- CR Robbins and MK Bahl, Analysis of hair by electron spectroscopy for chemical analysis, J Soc Cosmet Chem 35(8) 379-390 (1984)
- Y Masukawa, H Tsujimura H Tanamachi, H Narita and G Imokawa, Damage to human hair caused by repeated bleaching combined with daily weathering during daily life activities, Exog Dermatol 3(6) 273-281 (2004)
- T Habe, N Tanji, S Inoue, M Okamoto, S Tokunaga and H Tanamach, ToF-SIMS characterization of the lipid layer on the hair surface. I: The damage caused by chemical treatments and UV radiation, Surf Interface Anal 43(1-2) 410-412 (2011)
- ED Goddard and RB Hannan, Polymer/surfactant interactions, J Am Oil Chem Soc 54(12) 561-566 (1977)
- K Ohbu, O Hiraishi and I Kashiwa, Effect of quaternary ammonium substitution of hydroxyethylcellulose on binding of dodecyl sulfate, J Am Oil Chem Soc 59(2) 108-112 (1982)
- Miyuki Miyake, Property and function appearance of cationic compounds in hair surface modification, Hyomen 47(12) 433-445 (2009)
- PL Dubin, JH Gruber, J Xia and H Zhang, The effect of cations on the interaction between dodecylsulfate micelles and poly(ethyleneoxide), J Colloid Interface Sci 148(1) 35-41 (1992)
- K Yoshida, Y Morishima, PL Dubin and M Mizusaki, Binding of cationic mixed micelles to pyrene-labeled poly((acrylamido)-2-methylpropanesulfonate), Macromolecules 30(20) 6208-6214 (1997)
- M Miyake and Y Kakizawa, Morphological study of cationic polymer-anionic surfactant complex precipitated in solution during the dilution process, J Cosmet Sci 61(4) 289-301 (2010)
- Y Tsujino and M Miyake, Ionic Complex/Coacervate (first volume), Marcel 236 40-47 (2013)
- Y Tsujino and M Miyake, Relationship Between 'Shampoo' and 'Silicone' and 'Ion Complex,' Marcel 237 16-23 (2013)
- Y Tsujino, Technology to improve the smoothness of the hair surface in shampoo formulation, and its influence on hair-coloring and permanent-waving, J Japan Hair Science Association 47(4) 16-24 (2015)
- Y Iwasawa, Measure Dilution Ratio of Shampoo, Marcel 237 24-25 (2013)
- A Benninghoven, Secondary Ion Mass Spectrometry, John Wiley and Sons, New York (1987) p 671
- DH Kirby, The waving efficiency of cold permanent wave lotion, Drug Cosmet Ind 80(3) 314-397 (1957)
- K Sugibayashi, H Todo and S Itami, Scientific Investigation About Whether Silicone Becomes the Cause of Blocking of Skin Pores, Marcel 237 34-37 (2013)