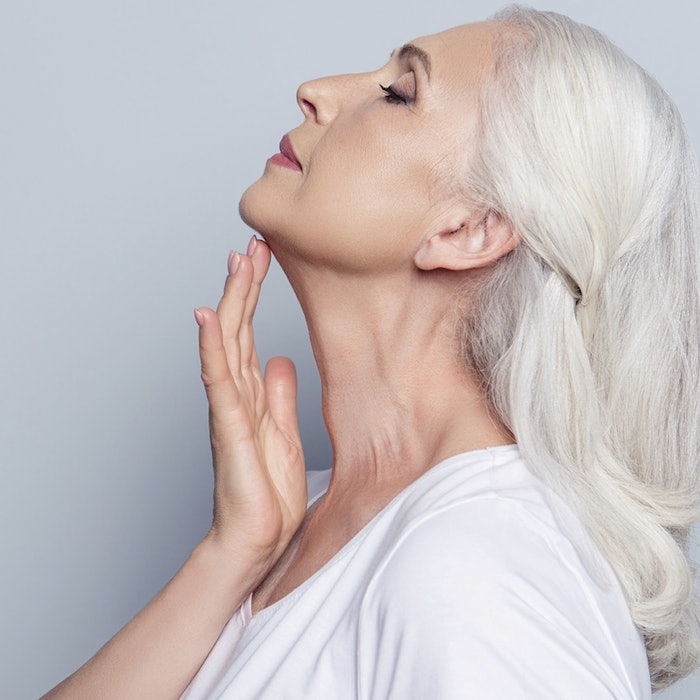
There's a famous line in the movie “Raiders of the Lost Ark” where Harrison Ford’s character, Indiana Jones, explains the poor state of his aging body as, “It’s not the years, it’s the mileage.” Despite Ford’s character being fictional, there is quite a bit of truth in this statement; and it directly applies to the challenges faced by the skin care industry as it seeks to maintain the youthful appearance of those who purchase its products. While the gradual passing of time itself can eventually age one's appearance, the repeated daily insults of solar irradiation and countless sources of toxic urban pollution, e.g., dust, high energy visible light, ozone and tobacco smoke, can rapidly accelerate the aging process and leave skin appearing decades older than its chronological age.
In efforts to prevent both premature skin aging and general effects of aging itself, the personal care industry has responded with a wide range of actives. Skin care products are now designed with materials that block UV rays. They also contain antioxidants, which can be effective against the reactive oxygen species formed from exposure to urban pollution. In addition, specialty ingredients have been developed to target specific effects of aging, such as a decline in collagen, elastin or hyaluronic acid production; or increases in entities including matrix metalloproteinases (MMPs), which promote the breakdown of essential extracellular matrix components.
Despite the overall effectiveness of these skin care actives, the skin will continue to age and with this aging comes an inevitable accumulation of senescent cells. Unfortunately, senescent cells are more than just cells that no longer replicate. In fact, an accumulation of senescent cells in any organ of the body is associated with a decline in the functioning of that organ. Also unfortunately, senescent cells can greatly accelerate the aging process by their presence alone.1
The skin is no exception to this observation. Therefore, countering the effects of senescent cells in the skin represents an excellent target for future active ingredients. However, before any sort of active can be developed, it is essential to understand how senescent cells can form, their basic characteristics and how they affect neighboring cells.
Cellular Senescence
In 1961, Leonard Hayflick and Paul Moorhead published a paper describing their discovery that human cells can only divide a finite number of times.2 This limit to the number of cell divisions a normal cell can make is now called the Hayflick Limit, and it introduced the concept of replicative senescence. Once a cell has reached replicative senescence, no amount of stimulation with growth factors will stimulate it proliferate again.
The molecular mechanism behind replicative senescence is thought to be associated with telomeres. Telomeres are tandem repeating DNA sequences (TTAGGG) found at the ends of linear DNA molecules.3 Telomeres are essential to the process of linear DNA replication; however, as part of the replication process, each time a cell copies its genomic DNA to undergo cell division it loses a portion of these telomeres. Once the telomere-repeats reach a shortened critical length, the cells can no longer undergo cell division and they enter replicative senescence.
Before utilizing senescent cells to test an active, it is essential to understand how senescent cells form, their basic characteristics and how they affect neighboring cells.
While replicative senescence can be thought of as a more chronological-based mechanism of aging, cellular stress can dramatically accelerate the onset of cellular senescence. This process is referred to as stress-induced premature senescence (SIPS), and often involves exposing cells repeatedly to insults that form reactive oxygen species such as peroxides4 or UV light.5 However, the direct generation of reactive oxygen species is not necessary to induce SIPS since other forms of cellular stress can damage DNA and induce senescence. These include: mitochondrial dysfunction,6 autophagy deficiency,7 cellular membrane disruption8 and exposure to chemotherapeutic agents.9 As with replicative senescence, once cells enter SIPS, they will no longer undergo the process of cellular replication.
Despite the various methods by which cellular senescence can be induced, in the end, the resulting senescent cells seem to share similar characteristics—the most important of which is the activation of the p53 pathway. This pathway functions to carefully monitor the integrity of cellular genomic DNA, since damage to the genomic DNA can potentially be tumorigenic. At low levels of DNA damage, the p53 pathway can be temporarily activated, which will, in turn, activate cell cycle inhibitors such as p21 and p16INK4a and stop the cell from replicating until the DNA damage is repaired. However, at a certain DNA damage threshold, the genomic damage is considered by the cell to be irreparable and the p53 pathway will push the cell to either a state of permanent growth arrest (senescence) or to a programmed cell death pathway (apoptosis) in an effort to prevent extensive, and potentially cancer-causing, DNA damage from being propagated by dividing cells.
In addition to the activation of the p53 pathway and subsequent expression of p21 and p16INK4a, senescent cells tend to display an interesting characteristic that makes them easy to visualize using a simple staining procedure. As cells become senescent, a certain lysosomal β-galactosidase experiences a shift in the pH at which it is active; rather than its typical activity at a pH of 4, the enzyme becomes active at a pH of 6. This shift has led to its referral as senescence associated β-galactosidase (SA β-gal), which is widely used to mark senescent cells within a larger field of cells viewed microscopically in order to determine the percentage of senescent cells in a sample. Additional characteristics all senescent cells appear to share include an increase in their size, the absence of proliferation markers, and changes in their heterochromatin.10
The accumulation of senescent cells within the skin can further accelerate the aging process.
Consequences of Senescent Cells
One of the most troubling aspects of senescent cells is they release a number of dangerous materials into their surrounding cellular environment. These materials can not only damage the local extracellular microenvironment, but also promote harmful effects such as inflammation, tumorigenesis and the conversion of otherwise healthy neighboring cells into senescent cells. It is through the release these dangerous materials that the accumulation of senescent cells can contribute to the decline in function of the skin and accelerate the aging process.
The mechanisms by which senescent cells release materials into their environment generally fall within two categories:
- The release of soluble factors via the senescent-associated secretory phenotype (SASP); or
- The release of more complex factors via extracellular vesicles (EV).
Research into these pathways could help to elicit details about how senescent cells impact the otherwise healthy cells around them, and may provide insight into how to develop skin care actives that can prevent or reverse the adverse effects of senescent cells.
Senescence-associated Secretory Phenotype
SASP appears to be a universal feature observed in all senescent cells11 and involves the chronic release of cytokines, i.e., IL-1α, IL-1β, IL-6, IL-8 and TNF-α; chemokines; growth factors including TGF-β and GM-CSF; and proteases such as MMP1 and MMP3.12, 13 Functionally, it is thought that the release of soluble factors through SASP is intended to recruit immune cells to senescent cells to facilitate their removal. However, studies have shown that the chronic release of soluble factors through SASP is enough to convert neighboring cells to the senescent phenotype and can also be potentially tumorigenic to neighboring cells.10 In addition, the prolonged release of inflammatory cytokines can lead to chronic inflammation, while the chronic release of proteases can further augment the decline in ECM components observed with aging.
While some of the components released via SASP have been described, the reality is the sheer variety of soluble factors released can be quite extensive and the listed materials are by no means a complete library. Also, despite the fact that more and more soluble factors released by senescent cells are being characterized, the work is far from complete and numerous others, along with their adverse effects on target cells, remain unknown.
Characterizing the release of exosomes can determine potential actives to prevent their adverse effects.
Fortunately, some analytical methods are well-suited for screening the soluble factors released via SASP. From a genetic perspective, DNA microarrays can easily determine which genes for cytokines, chemokines, growth factors and proteases are being transcribed by senescent cells. Since most DNA microarray chips encompass a significant portion of the human genome, they may provide a means to best capture a complete picture of which soluble factors are present. Alternatively, commercially available protein arrays or multiplexing, bead-based enzyme-linked immunosorbent assays (ELISA) also can be used to quantify and characterize which soluble factors are being released at the protein level by senescent cells into the environment. While protein arrays and multiplexing ELISA-based assays have a considerably smaller range than DNA microarrays, they are none the less quite effective for gaining insight into complex mixes of soluble factors.
Extracellular Vesicles
In addition to the release of soluble factors, senescent cells communicate with neighboring cells through the release of extracellular vesicles. While extracellular vesicles can range in size and cellular source, particular interest has emerged in exosomes. Exosomes are small extracellular vesicles (30–100 nm) that originate as intraluminal vesicles in the late endosomal compartment of cells. They are released when the vesicle containing them fuses with the plasma membrane. Once thought of as cellular trash receptacles, they are now known to be a form of normal communication between cells and contain a variety of materials including mRNA, miRNA, genomic and mitochondrial DNA, proteins and lipids. While the cellular release of exosomes is a normal process, exosome release is greatly increased in senescent cells via a p53-dependent mechanism potentially as a means to remove damaged DNA.12 Unfortunately, exosomes released by senescent cells and taken up by neighboring cells can cause senescence in the neighboring cells,14 and/or promote an inflammatory response,15 or can even become tumorigenic.12
As can be imagined, the contents of exosomes released by cells are far from homogeneous and can vary extensively. Following in the trend of soluble factors, there is a need to characterize the exosomes released by senescent cells in order to determine how they impact neighboring cells and whether it is possible to target them using actives to prevent any adverse effects they may promote. Of particular interest is the RNA cargo, since both the mRNA and the miRNA contained within the exosome released by the senescent cell will be functional when taken up by a normal neighboring cell. Thus, the mRNA transcribed within the senescent cell can be translated by the neighboring cell taking up the exosome and likewise, the miRNA produced within the senescent cell can exert a regulatory effect within the receiving cell.
There are multiple ways to induce cellular senescence in the lab that can mimic the way senescent cells are formed in vivo.
For some time, the only way to recover exosomes for analysis was using an ultracentrifuge to pellet them. Most labs do not have such a specialized piece of equipment. However, methods are now available to recover exosomes via precipitation or filtration, which require nothing more than centrifuges commonly used for cell culture practice. And once an enriched collection of exosomes is gathered, microarray-based RNA analysis may be a good way to determine the species of RNA present in the exosome samples. Traditional DNA microarrays actually measure mRNA, so DNA microarray characterization of exosomes would provide details on which mRNA species are being exported by senescent cells. In addition to traditional DNA microarrays, commercially available human miRNA arrays are available that contain extensive miRNA libraries, which would be extremely useful for determining the miRNA species produced by senescent cells.
While there is keen interest in the RNA components of exosomes, exosomes also contain proteins and lipids. Thus, the protein content of exosomes can be determined using traditional protein array and Western blotting analyses while the lipid content can be measured using traditional lipid composition assays. It also should be noted that both the protein and lipid components of the exosome may contain active forms of signaling pathways that would exert an effect in the target cells taking them up. All in all, exosomes provide a broad and challenging range of targets for skin care actives to potentially treat the effects of senescent cells in skin.
Concluding Remarks
The accumulation of senescent cells within the skin can further accelerate the aging process, leading to a decline in both the function and appearance of skin. Fortunately, from a research perspective, there are multiple ways to induce cellular senescence in the lab that can mimic the way senescent cells are formed in vivo. The availability of these models, coupled with new array-based analytical techniques that can rapidly screen thousands of cellular components, will ultimately lead to a better understanding of senescent skin cells and the development of new actives to further delay the effects of aging in the skin.
References
- DJ Baker et al, Naturally occurring p16(Ink41)-positive cells shorten healthy lifespan, Nature 530 184–189 (2016)
- L Hayflick and PS Moorhead, The serial cultivation of human diploid cell strains, Exper Cell Res 25 585–621 (1961)
- CB Harley, AB Futcher and CW Greider, Telomeres shorten during aging of human fibroblasts, Nature 345 458–460 (1990)
- P Dumont et al, Induction of replicative senescence biomarkers by sublethal oxidative stresses in normal human fibroblasts, Free Rad Biol and Med 28(3) 361–373 (2000)
- F Chainiaux et al, UVB-Induced premature senescence of human diploid skin fibroblasts, Intl J of Biochem and Cell Biol 34 1331–1339 (2002)
- CD Wiley et al, Mitochondrial dysfunction induces senescence with a distinct secretory phenotype, Cell Metabolism 23(2) 300–314 (2016)
- X Song et al, Autophagy deficient keratinocytes display increased DNA damage, senescence and aberrant lipid composition after oxidative stress in vitro and in vivo, Redox Biol 11 219–230 (2017)
- WK Martins et al, Membrane damage by betulinic acid provides insights into cellular aging, Biochemica et Biophysica Acta 1861 (1Pt A) 3129–3143 (2017)
- UK Rajarajacholan and K Riabowol, Aging with ING: A comparative study of different forms of stress induced premature senescence, Oncotarget 6(33) 34118–34127 (2015)
- JP Magalhaes and JF Passos, Stress, cell senescence and organismal aging, Mech of Aging and Dev [Epub ahead of print] (2017)
- HO Byun et al, From cell senescence to age-related diseases: Differential mechanisms of action of senescence-associates secretory phenotypes, BMB Reports 48 (10) 549–558 (2015)
- M Takasugi, Emerging roles of extracellular vesicles in cellular senescence and aging, Aging Cell [Epub ahead of print] (2017)
- GC Leonardi et al, Aging: From inflammation to cancer, Immunity and Aging [Epub ahead of print] (2018)
- AHJ AL-Mayah et al, Exosome-medicated telomere instability in human breast epithelial cancer cells after x irradiation, Radiation Res 187 98–106 (2017)
- Z Wang and PM Lieberman, The crosstalk of telomere dysfunction and inflammation through cell-free TERRA containing exosomes, RNA Biol13, 690–695 (2016)