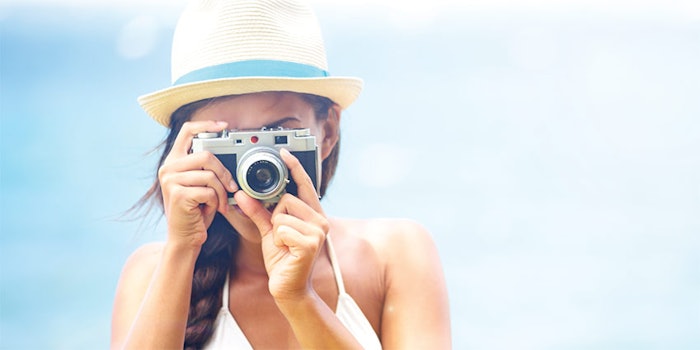
Despite abundant sunscreen offerings, consumers still unintentionally expose poorly protected skin to damaging UV radiation. While they understand SPF and UVA values on sunscreen packaging, insufficient knowledge of how these values are calculated can lead to misuse. Such measurements are obtained using validated and standardized methods and are controlled under strict laboratory conditions by trained technicians.
However, this data does not take actual-use conditions into consideration.1, 2 In fact, studies show that consumers apply significantly less quantities than are used for test methods. Labels direct users to “apply generously,” but the definition of generous will vary from person to person. Sun care brands, aware that insufficient quantities of sunscreen will be used, insist heavily on reapplication every two hours, especially after bathing. Yet, if such advice is rarely followed during beach use, it is almost never respected with daily-wear facial sunscreens.3
Besides quantity, quality of application is also an issue. Some areas of the body are more difficult to reach than others and are neglected, which can lead to severe sunburn on unprotected zones.4-6 Furthermore, hasty application can lead to poor coverage and a heterogeneous film.
To help physicians and dermatologists analyze and visualize skin pigmentation and sun damage—and reinforce to the public the importance of sun protection—UVA cameras have been used.7-9 Building from this approach, the present work examines the utility of such cameras in a novel application: to quantify the performance of UV protection.10, 11
Here, an adapted UVA camera was employed to evaluate the quality and evenness of sunscreen applied to test subjects, as well as how the photoprotective films evolved over the course of six hours. These results provided visual evidence in support of the reapplication of sunscreens.
In addition, the authors demonstrated how formulators might apply this technique to boost the performance of sunscreen films using select ingredients. A natural wax derivative is provided as an example.
Equipment Setup
Since standard equipment for the desired tests was unavailable commercially, a UV acquisition system was developeda for the present work (see Figure 1).12 Following in-depth analysis of the absorption spectra of different sunscreens, the UVA wavelength band was chosen (see Figure 2) as the most suitable to highlight the presence of product on the skin. The initial infrared (IR) cut filter of a camerab based on a high-resolution sensor with a size of 14.9 × 22.3 mm and a full resolution of 24.23 mega pixels (Mp) (6,024 × 4,022 pixels), was removed and replaced with a specific clear filter, making the sensor very sensitive to UV
(see Figure 3).
In order to acquire images only within the UV absorbance band, a specific UV bandpass filter with the useful range from 335-400 nm and 85% peak transmission at 365 nm was integrated in front of the sensor. Notably, this acquisition module was optimized for wavelength acquisition below 400 nm and could not be used for RGB color image capturing.
To ensure standardization of the acquisition, an illumination module composed of four UVA light bulbsc (see Figure 2) was installed. This light source emits wavelengths between 350-400 nm. The illumination module was divided into two parts placed on either side of the acquisition system, to ensure homogeneity of lighting over the entire surface of the face. A warm-up time of at least 10 min was necessary to ensure good lighting stability. The specifications of the bulbs used allowed for full compatibility with clinical relevance.
To ensure correct repositioning of volunteers at different acquisition time points, a specific support was designed to maintain their faces in the same position. This support also enabled the capture of images at three distinct positions, -45 degrees, 0 degrees and 45 degrees, based on an innovative indexed rotation system. Moreover, based on its distinct design, the support made it possible to acquire a complete facial image without any chin or forehead support; only ear supports. Within an image series, a live video mode with ghost overlays of the first acquisition, together with a screen display of a vertical line and a horizontal line, allowed for easy and consistent realignment of the inclination of a subject’s face.
The described UV camera is capable of easily visualizing both the location and uniformity of sunscreen application.
The acquisition system was connected to a laptop via a USB link. Dedicated software supported fine adjustments and the optimization of acquisition parameters to obtain the best dynamics and minimize the signal-to-noise ratio. Last, the software used was capable of managing acquisitions in a clinical study of multiple volunteers over multiple visits.
Image Analysis
The grayscale images generated were then analyzed to determine the UV absorption of the applied sunscreen products. Darker regions corresponded to higher UV absorption, while lighter regions indicated lower absorption. The level of UVA protection was thus linked directly to the intensity of the gray level.
By defining a precise region of interest on the image, it was possible to extract quantitative results for sunscreen UVA absorption.
In order to quantify the durability of a formula, a parameter R was determined for a given analysis area. This R value represented the average gray level of an area, scaling from small values of R for dark areas to large values for light areas.
Before applying sunscreen, Caucasian skin appeared light, generating large R values. After sunscreen application to given area of skin, said area appeared darker, giving small R values. As the sunscreen film wore off over the course of the study period, this area became progressively lighter and the R value increased. Remanence was thus evaluated by the change in R over time, with greater remanence corresponding to smaller increases in R. Statistical analysis was carried out to determine the significance of the evolution of the R parameter. Each time point was compared with T0.
Figure 4 shows the durability over time of a sunscreen with an SPF 30 and UVA-PF 15 (in silico).
Continue reading in the April 2019 Digital Edition...
References
- Diffey, B., et al. (2017). Labelled sunscreen SPFs may overestimate protection in natural sunlight. Photochem Photobiol Sci, 16.
- Pissavini, M., et al. (2012). Predicting the efficacy of sunscreens in vivo veritas. Intl J Cos Sci, 34, 44-48.
- De Villa, D., et al. (2010). Reapplication improves the amount of sunscreen, not its regularity, under real life conditions. Photochem and Photobio, 87, 457-60.
- Jovanovic, Z., et al. (2017). Conventional sunscreen application does not lead to sufficient body coverage. Int J Cosmet Sci, 39, 550-555.
- Lott, D., et al. (2003). Uniformity of sunscreen product application: A problem in testing, a problem for consumers. Photodermatol, Photoimmunol and Photomed, 19, 17-20.
- Ou-Yang, H., et al. (2017). Sunburn protection by sunscreen sprays at beach. Cosmetics, 4, 10.
- Mahler, H., et al. (2007). Long-term effects of appearance-based interventions on sun protection behaviors. Health Psych, 26(3) 350-360.
- Pratt, H., et al. (2017). UV imaging reveals facial areas that are prone to skin cancer are disproportionately missed during sunscreen application. PloS One.
- Fulton, J. (1997). Utilizing the ultraviolet (UV Detect) camera to enhance the appearance of photodamage and other skin conditions. Dermatologic Surgery, 23, 163-9.
- Crowther, J. M. (2018). Understanding sunscreen SPF performance using cross-polarized UVA reflectance photography. Int J Cosmet Sci, 40, 127-133.
- Gálvez, M., et al. (2018). Time required for a standard sunscreen to become effective following application: A UV photography study. J Eur Acad Dermatol Venereol, 32, e123-e124.
- Zucco, M., et al. (2015). A hyperspectral camera in the UVA band. IEEE Transactions on Instrumentation and Measurement, 64(6), 1425-1430.