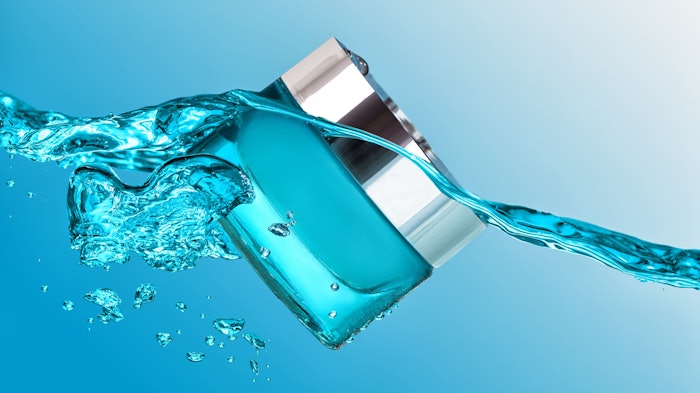
Editor's Note: This work examines the potential of water, treated via an ultrafine bubble technology, to deliver actives into skin. The penetration of niacinamide and carnosine (peptide) from a cosmetic formula is evaluated. Additional applications are proposed.
Log in to view the full article
Editor's Note: This work examines the potential of water, treated via an ultrafine bubble technology, to deliver actives into skin. The penetration of niacinamide and carnosine (peptide) from a cosmetic formula is evaluated. Additional applications are proposed.
The cosmetics industry is rapidly evolving, driven by consumer demand for innovative, highly effective, natural and sustainable products.1 Recent trends show a continued shift toward clean labels and natural ingredients, for which researchers are exploring novel active compounds from plant extracts, marine sources and biotechnology. These efforts have led to advancements in anti-aging, skin brightening and protective formulations, among others.
Amidst this innovation, water — the most prevalent ingredient in many cosmetic formulations — remains understudied. A search in major cosmetic science journals reveals a scarcity of research on water's role in cosmetic products, despite its fundamental importance in product stability, ingredient delivery and overall efficacy.
One promising area of innovation is the application of ultrafine bubbles (UFBs) in cosmetic formulations. These gas-filled spheres have diameters less than one micron, typically between 100-300 nm2. They were recognized by ISO in 2017 (ISO 20480-1), and they possess unique properties that offer interesting possibilities for cosmetic applications.
These properties include:
- a high surface-to-volume ratio, which enhances surface activity, enabling efficient mass transfer and improved gas solubility;3-5
- good stability due to negative electrostatic charge and reduced buoyancy, prolonging their lifespan in liquids;6-8 and
- improved penetration and permeability, thanks to their small size, which makes them ideal carriers for active ingredients.9-11
Researchers have proposed12 an “attract and deliver” hypothesis for UFB-enhanced skin permeation. That is, the bubbles may attract and aggregate active ingredients through electrostatic and non-polar forces at the gas-liquid interface of the bubble, potentially shuttling these ingredients deeper into the skin. Such a dynamic could reduce reliance on exogenous chemical penetrating agents that may irritate sensitive skin.
The present work explores the potential of UFB-treated watera as an active delivery vehicle in cosmetic applications. Test formulas are prepared to examine its effects on the penetration of niacinamide and carnosine (peptide) into skin. Additional applications are also proposed.
Niacinamide Penetration and Skin Hydration Ex vivo
Skin permeation: Ex vivo testing of the UFB technology was conducted using confocal Raman spectroscopy to visualize the permeation of target molecules into the stratum corneum (SC) and viable epidermis. Skin samples (1 cm in diameter) were treated with 40 mg of two test formulas that were identical except for their water: one incorporated DI water and the other, UFB-treated water (see Table 1).
The formulas were massaged onto skin samples with a glass rod for 60 sec. These samples were placed in a Franz diffusion cell (filled with DI water) and incubated for 2 hr at 32°C. Excess formula was then removed via Kimwipe before Raman analysis (see Figure 1 schematic).
Figure 2 shows the results of the niacinamide test, visualized via confocal Raman microscopy. These showed that Formula 1, with deionized (DI) water, was not successful in delivering niacinamide into the SC – the active remained on the skin surface. In contrast, Formula 2, with the UFB-treated water, showed significant penetration of the niacinamide through the SC and extending into the viable epidermis.
The mechanism by which the UFB water could enhance the dermal penetration of active ingredients such as niacinamide is still under investigation. It is likely via its function as a nano-scale carrier, considering the sub-200 nm size of the bubbles and characteristic Brownian motion kinetics. However, additional research is required.
Skin hydration: Figure 3 shows the same niacinamide-containing formulations on the skin, but now focusing on the O-H frequency of water in the Raman spectrum to visualize skin hydration.
The Raman spectral band corresponding to O-H stretching vibrations (3350-3550 cm-1) indicates the presence of hydroxyl-containing molecules. As water (H2O) represents the predominant hydroxyl-containing species in biological tissue, the intensity of this band can be used to assess the relative water content and hydration status in skin. However, it should be noted that other hydroxyl-containing biomolecules may contribute to this spectral region, albeit to a much lesser extent than water, due to their lower relative concentrations.
Quantitative analysis of the water content within the skin samples showed that Formula 2, containing UFB-treated water, resulted in significantly higher hydration levels on the surface and viable epidermis in comparison with Formula 1, containing DI water.
What’s more, Formula 2 was tested 26 months after initial analysis and the same niacinamide delivery benefits and increased hydration were observed, highlighting the stability of formulas incorporating UFB-treated water (not shown).
Carnosine Penetration Ex vivo
Ex vivo studies also were conducted using HPLC analysis to measure the permeation of the dipeptide carnosine into skin. Skin samples (1 cm in diameter) were treated with two identical test formulas except for their water – again, one included DI water and the other, UFB-treated water. Forty milligrams of each formula was massaged into skin samples with a glass rod for 60 sec. The samples were then placed in a Franz diffusion cell and incubated for 24 hr at 32°C.
As Figure 4 shows, the cumulative penetration of carnosine was more than 24 mg/mL after 24 hr with the UFB treatment, compared with only 8 mg/mL with the control.
Discussion
Initial research of the UFB technology provides encouraging results and only recently has it been more widely incorporated into cosmetics and personal care applications.10, 16 Active ingredient selection remains an ongoing area of study, especially in consideration of the structural moieties and functional groups that are well-tolerated by UFBs.
However, the technology has shown compatibility with most existing cosmetic formulations and stability under extreme high and low temperatures – down to 0°C17 and up to autoclave sterilization cycles at 121°C under 15 psi for 15 min; it also tolerates high shear forces during mixing (not shown).
Since the mechanics of the UFB technology are still being explored, testing plays a crucial role in identifying the optimal concentration of UFBs for the given formulation, combination of active ingredient(s) and UFB parameters (i.e., size, concentration, gas composition, etc.) to maximize the desired benefits and outcomes of personal care formulations.
Interestingly, gas selection presents an exciting avenue for exploration. The current UFBs use ambient air (primarily nitrogen and oxygen), but future possibilities could incorporate gases chosen for their cosmetic benefits; oxygen, nitrogen, carbon dioxide, hydrogen and argon are being investigated.
Another area of focus is ensuring comfort for users. Notably, UFB formulations have been positively received by consumers without reported adverse reactions (not shown). To further ensure safety, the UFB technology has been self-GRAS certified through a third-party clinical research organization. In addition, studies exploring the interactions of UFBs with skin, their impact on skin health and their relationship with the skin microbiome are in progress to further optimize their benefits for skin care applications.
For example, UFBs could potentially enhance the delivery of hydrating ingredients such as hyaluronic acid or glycerin, or anti-aging actives like peptides, vitamins or antioxidants, amplifying their effects on skin renewal and collagen production.13 Cleansers and makeup removers with UFBs may offer gentler yet effective cleansing by breaking down and lifting away impurities without the need for surfactants, potentially reducing skin irritation.14
Moreover, UFBs may help to stabilize emulsions and suspensions, reducing the need for additional stabilizers while extending shelf life.15 This aligns well with the growing consumer demand for clean and minimalist formulations, contributing to more sustainable product development and potentially reducing waste. From a business perspective, the technology can also be integrated for on-site UFB water generation.
Conclusions
As shown here, ultrafine bubblesa present a novel opportunity for the cosmetics and personal care industry. They offer a unique combination of benefits that addresses many current industry challenges (i.e., actives delivery, stability) and consumer demands for more sustainable and green alternatives. Further research will uncover their greater potential.
Thus, the described UFB technology provides formulators with a new tool to create highly effective, environmentally friendly products that meet evolving consumer expectations. This technology shows great potential to be the next significant advancement in cosmetic science, enabling the development of cutting-edge products that combine performance, safety and sustainability.
a Hydrosome H2O is a patented technology of Hydrosome Labs.
References
1. Mayo, A. (2021, Oct 18). 2030 Glow-up: The future of clean beauty. NIQ. Available at https://nielseniq.com/global/en/insights/analysis/2021/2030-glow-up-the-future-of-clean-beauty/
2. Foudas, A.W., Kosheleva, R.I., Favvas, E.P., Kostoglou, M., Mitropoulos, A.C. and Kyzas, G.Z. (2023). Fundamentals and applications of nanobubbles: A review. Chemical Engineering Research and Design, 189 64-86; https://doi.org/10.1016/j.cherd.2022.11.013.
3. Azevedo, A., Etchepare, R., Calgaroto, S. and Rubio, J. (2016). Aqueous dispersions of nanobubbles: Generation, properties and features. Minerals Engineering, 94 29-37; https://doi.org/10.1016/j.mineng.2016.05.001.
4. Javed, M., Matloob, A., Ettoumi, F., Sheikh, A.R., Zhang, R. and Xu, Y. (2023). Novel nanobubble technology in food science: Application and mechanism. Food Innovation and Advances, 2(2) 135-144; https://doi.org/10.48130/FIA-2023-0014.
5. Temesgen, T., Bui, T.T., Han, M., Kim, T. and Park, H. (2017). Micro and nanobubble technologies as a new horizon for water-treatment techniques: A review. Advances in Colloid and Interface Science, 246 40-51; https://doi.org/10.1016/j.cis.2017.06.011.
6. Brenner, M.P. and Lohse, D. (2008). Dynamic equilibrium mechanism for surface nanobubble stabilization. Phys. Rev. Lett., 101(21) 214505; https://doi.org/10.1103/PhysRevLett.101.214505.
7. Kim, E., Choe, J.K., Kim, B.H., Kim, J., Park, J. and Choi, Y. (2020). Unraveling the mystery of ultrafine bubbles: Establishment of thermodynamic equilibrium for sub-micron bubbles and its implications. J Colloid and Interface Science, 570 173-181; https://doi.org/10.1016/j.jcis.2020.02.101.
8. Nirmalkar, N., Pacek, A.W. and Barigou, M. (2018). On the existence and stability of bulk nanobubbles. Langmuir, 34 (37) 10964-10973; https://doi.org/10.1021/acs.langmuir.8b01163.
9. Ito, M. and Sugai, Y. (2021). Nanobubbles activate anaerobic growth and metabolism of Pseudomonas aeruginosa. Sci Rep, 11(1) 16858; https://doi.org/10.1038/s41598-021-96503-4.
10. Park, Y., Shin, S., Shukla, N., Kim, K. and Park, M.-H. (2022). Effects of nanobubbles in dermal delivery of drugs and cosmetics. Nanomaterials, 12(19) 3286; https://doi.org/10.3390/nano12193286.
11. Wang, X., Yuan, T., Lei, Z., Kobayashi, M., Adachi, Y., Shimizu, K., Lee, D.-J. and Zhang, Z. (2020). Supplementation of O2-containing gas nanobubble water to enhance methane production from anaerobic digestion of cellulose. Chemical Engineering Journal, 398 125652; https://doi.org/10.1016/j.cej.2020.125652.
12. Hydrosome Labs. (Accessed 2024, Nov 22). Advancing ultrafine bubble technology. Available at https://hydrosomelabs.com/.
13. Jin, J., Yang, L., Chen, F. and Gu, N. (2022). Drug delivery system based on nanobubbles. Interdisciplinary Materials, 1(4) 471-494; https://doi.org/10.1002/idm2.12050.
14. Zhu, J., An, H., Alheshibri, M., Liu, L., Terpstra, P.M.J., Liu, G. and Craig, V.S.J. (2016). Cleaning with bulk nanobubbles. Langmuir, 32 (43) 11203-11211; https://doi.org/10.1021/acs.langmuir.6b01004.
15. Shen, Y., Longo, M.L. and Powell, R.L. (2008). Stability and rheological behavior of concentrated monodisperse food emulsifier coated microbubble suspensions. J Colloid and Interface Sci, 327(1) 204-210; https://doi.org/10.1016/j.jcis.2008.07.047.
16. Lee, S.-J., Lee, S.-H. and Park, S. (2020). A study on skin permeability enhancement of active substances in cosmetics using nanobubble technique. J Korean Applied Sci and Tech, 37(4) 1041-1051; https://doi.org/10.12925/JKOCS.2020.37.4.1041.
17. Cho, C.-H., Shin, H.-J., Singh, B., Kim, K. and Park, M.-H. (2023). Assessment of sub-200-nm nanobubbles with ultra-high stability in water. Appl Water Sci, 13(7) 149; https://doi.org/10.1007/s13201-023-01950-1.