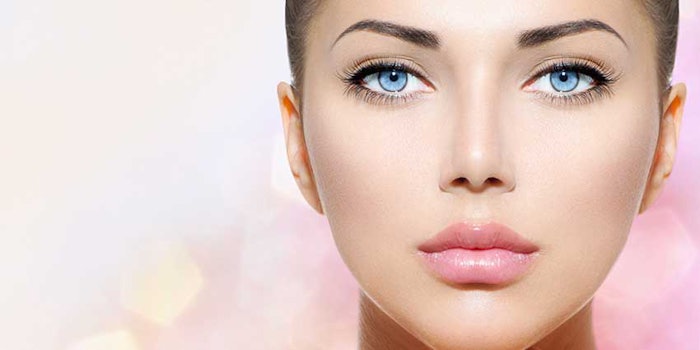
Editor’s note: The first part of this article appeared in November 2011 and reviewed normal human floral of the skin. This installment focuses on the ocular area.
Log in to view the full article
Editor’s note: The first part of this article appeared in November 2011 and reviewed normal human floral of the skin. This installment focuses on the ocular area.
As stated in the first part of this article, cosmetics are not expected to be sterile; however, they must be produced under Good Manufacturing Practices (GMP) and be free from microorganism concentrations and types that may cause product spoilage or adversely impact consumer health. Humans are host to an extensive assortment of microorganisms, and while the normal flora play an essential role in health, some may also become pathogenic under certain conditions, e.g., if the microorganisms gain access to deeper tissues as a result of trauma, surface breaks and wounds. The normal flora may also become pathogenic if transferred to immunocompromised persons. Therefore, preservative systems must be effective against flora transmitted from consumer handling and product application.
Like skin flora, the composition of normal eye flora is dynamic and can vary with age, environment, geographic region, exposure to antimicrobial agents, and immunological states.39–43 As knowledge of the normal ocular flora grows, the role and composition of personal care products and cosmetics may also diversify. For instance, saturated cleansing pads marketed for eyelid hygiene or cleansing must provide antibacterial efficacy against eyelid bacteria but without causing irritation; thus, while broad-spectrum protection may be warranted, perhaps preservatives may be more tailored to uniquely match the insult challenges of this specific site. Taking all this into consideration, the present article reviews not only the transient and resident microbes of ocular flora, but also considers how cosmetic products may affect and/or be impacted by them.
Ocular Flora
As previously stated, the human eye area harbors microbial flora that plays an essential role in both the prevention and cause of ocular infection.43 Normal ocular flora plays a protective role by maintaining homeostasis through: secretion of antimicrobials; release of chemical mediators, e.g., proteases and peptides, which may impact inflammatory and immune response; outcompeting ocular pathogens for available nutrients; preserving epithelial integrity; and inhibiting apoptosis.43 The external eye area and its surrounding tissue are host to several species of bacteria.44 Table 3 lists commonly isolated microbes. Generally, recovered densities from the normal conjunctiva are limited to fewer than 10 colonies on culture, whereas in an infectious state, confluent growth may be observed.45
Conjunctiva and eye area: Microorganisms cultured from the conjunctival sac and eye area include coagulase-negative Staphylococcus species, S. aureus, S. epidermidis, Cornyebacterium species, Streptococcus species (including S. pneumoniae), a variety of Gram-negative Bacillus species, P. acnes and fungi including Aspergillus species, Candida species, Penicillium species, Cladosporium species, Rhodotorula species and Alternaria species.43, 44, 46 Opportunistic pathogens such as Pseudomonas aeruginosa and Haemophilus influenzae may also be found.43 Klebsiella pneumoniae and Serratia marcescens, also both opportunistic pathogens, are considered occasional or transient flora.46, 47 Factors that enable these microorganisms to colonize the ocular surface without causing infection remain under investigation; however, an intact surface epithelium coupled with specific immune system regulatory patterns appears to be of prime importance.43
Eyelids: The bacterial flora of the eyelids resembles that of the conjunctiva. Typically, higher densities of bacteria are isolated from the eyelids than from the conjunctiva, with the normal eyelid almost always being culture-positive for bacteria. Coagulase-negative staphylococci tend to be the most prevalent eyelid isolate (generally exhibiting heavy growth on culture), followed by P. acnes, diphtheroids, S. aureus and S. viridans. A variety of Gram-negative bacteria have also been isolated from eyelids, but less frequently and at lower densities (i.e., only a few colonies) than the previously mentioned species.45
Recently, conjunctival microbiota were characterized by 16S rRNA analysis in a study by Dong et al., wherein sequencing of conjunctival rDNA from swabs of four healthy subjects showed the presence of 221 species-level phylotypes per subject, which were classified into 59 genera. Twelve genera— Pseudomonas, Propionibacterium, Bradyrhizobium, Corynebacterium, Acinetobacter, Brevundimonas, Staphylococci, Aquabacterium, Sphingomonas, Streptocococcus, Streptophyta, and Methylobacterium—were ubiquitous in all subjects and considered to be the primary conjunctival microbiota, including a mix of environmental, commensal and opportunistic pathogens. A substantial number of DNA reads (31%) belonged to unclassified or novel bacteria.48
Changes with Age
Initial colonization: The composition of normal ocular flora changes over the course of a human life. Initial ocular colonization patterns begin at birth, and differences have been noted in the microbial flora of babies born via caesarian section verses vaginally.43 Though coagulase-negative Staphylococcus species are the most commonly isolated bacteria, the conjunctiva of infants born vaginally have been shown to bear flora more like that of the vaginal area,49 whereas babies delivered by caesarian section harbor more skin-associated flora.49, 50 An infant’s own skin, respiratory tract and gastrointestinal flora are also important new sources of bacteria for the conjunctiva during the first days of life, regardless of delivery method. After birth, prior to prophylaxis, a dramatic increase in the frequency of culture-positive conjunctivas has been observed—from as few as 13% of newborns to 98% of 3- to 5-day-old infants, in one particular study.45
Studies by Hua et al. provide further evidence that the normal conjunctival flora is established during the initial months of life. In a cross-sectional study of 109 healthy babies ages 1 to 4 months, conjunctival swabs from both eyes showed a 23.9% positive culture rate consisting of 44 strains of ocular bacterial isolates. Of these isolates, 25 strains were Gram-positive cocci, 18 strains were Gram-positive bacilli, and one strain was a Gram-negative bacillus. Identified species included diphtheroid bacillus (unspecified, 38.6%), S. epidermidis (36.4%), and S. aureus (13.6%). The two Staphylococcus species coexisted with the diphtheroid bacillus in eight of 36 eyes. Results showed that gender, age, birth weight, gestational age and feeding form (i.e., breast, formula or mixed) had no correlation with conjunctival flora in these healthy infants.41
Species, density changes: Thiel and Schumacher conducted a study of 135 normal 3- to 90-year-old subjects over an 18-month period and found that the spectrum of aerobic bacteria colonizing the conjunctiva diversified and increased in density with age. As age increased, aerobic cocci were found less frequently, in favor of corynebacteria. Additionally, the proportion of anaerobic cocci such as P. acnes increased among the anaerobes.51 However, Propionibacterium species were most common in younger subjects and their prevalence decreased with age;45, 51 Table 4 provides an overview of bacterial isolation percentages from the conjunctiva by subjects’ age, from selected clinical studies.
It has been speculated that changes in ocular flora composition with age may be related to age-related goblet cell changes, lipid dysregulatory states and interactions of aqueous tear deficiency.51 Seborrheic blepharitis, which has been attributed to P. acnes, among other microorganisms, is more common in older adults. Its pathogenesis has been associated with increases in dry eye syndrome,52 which is more prevalent in those 40 and older and has been linked to age-related meibomian gland deficiency.53
Changes With Environment
Though the normal ocular flora is relatively consistent among human populations, geography and environment also influence its composition.43 Normal conjunctival flora found in rural populations differ from that found in developed world populations. In a study of 276 healthy residents of rural Masungbo, Sierra Leone in northern Africa, a high prevalence of ocular fungi and Gram-negative bacilli was found. The most commonly isolated microorganisms were coagulase-negative Staphylococcus species (28.6%), fungi (26.0%), S. aureus (19.9%), Gram-negative bacteria other than Pseudomonas and Haemophilus (9.8%), Nocardia/Actinomyces (6.5%) and Pseudomonas aeruginosa (6.2%).42 In this study, a number of participants were palm-tree tappers and subsistence farmers with frequent job-related ocular exposures and the authors suspect the colonizing microorganisms may have played a role in corneal infection after minor eye traumas.43 Tomar et al. also found a 6% recovery rate of P. aeruginosa from normal conjunctivas in a study carried out in India.54
In a 135-subject study in Germany, Megasphaera elsdenii, Bacteroides ureolyticus, Bacteroides pneumosintes, Stomatococcus mucilaginosus and group ANF (absolute non-fermenter) Corynebacterium strains were isolated for the first time in the eye area, indicating that what is considered normal eye flora may be geographic population-specific. For instance, ANF Corynebacterium was found so often that it was ascribed the role of an aerobic indicator organism in the study.51 In addition, in a southern Florida study, Candida parapsilopsis was the most frequently isolated fungal species from the normal external eye.55
In relation, Van Bijsterveld and Richards swabbed the eyes of 20 healthy subjects working in a horse stable. Results showed the presence of seven strains of Bacillus species, including Bacillus cereus, B. megaterium and B. coagulans.56, 57 These species are commonly found in soil, hay, horse manure and dust.56–58 Locatcher-Khorazo and Seegal also reported the presence of B. cereus in 1.5% of conjunctivas and eyelids of 40 to 90-year-old subjects.59 This microorganism may have been introduced from soil during outdoor activities such as gardening or from dust or hay in the environment.
Changes With Immunity
As would be expected, the composition of normal ocular flora changes with varying levels of immunity, which was shown in a study of hospitalized and immunocompromised patients and those having severe burns.55 In a four-month period, Pramhus et al. evaluated the eyes of 53 patients having severe thermal burns and observed a dramatic shift of the normal conjunctival flora—i.e., from S. epidermidis and Corynebacterium species, to S. aureus and Gram-negative bacilli. The shift commonly occurred on the fifth to sixth day post-burn and likely represented colonization of the conjunctiva by bacteria recovered from other sites in the body. Despite the high incidence of Pseudomonas aeruginosa isolates (34%), only three eyes in two patients developed infectious corneal ulcers.60
Direct exposure, superficial abrasions: Devices such as contact lenses can also influence normal ocular flora. It has been shown that in non-contact lens wearers, the cornea is extremely resistant to infection. The corneal stroma is protected by a multilayered epithelium and an associated basement membrane. In order for opportunistic pathogens such as P. aeruginosa to gain access to the corneal stroma and establish infection, a corneal epithelium breach (e.g., from minor eye trauma) must occur.61
In a study of 126 healthy subjects, ages 14 to 32, changes in conjunctival flora were observed before and after contact lens wearing during three, six and nine months. Prior to contact lens wearing, the following microorganisms were isolated: S. epidermidis (40.03%), Corynebacterium xerosis (2.78%), Corynebacterium acne (0.79%) and Bacillus subtilis (3.17%). After six and nine months of contact lens wearing, subjects showed a statistically insignificant decrease in conjunctival flora, although after contact lens wear, several new microorganisms appeared in the flora. The presence of the pathogens S. aureus (2.38%), Klebsiella pneumoniae, (1.59%) and Streptococcus viridans (0.79%) was detected in the conjunctival flora after six and nine months of contact lens wearing, indicating that microorganisms adhering to the contact lens may subsequently disseminate into and colonize the conjunctiva, which could increase the potential for infection. Contact lens wearers may have developed superficial abrasions in the corneal epithelium that offered entry for these microorganisms.44 Pathogens were also believed to have been introduced from contaminated contact lenses, lens cases or lens care solutions.44 Therefore, preventive steps should be taken to reduce the risk of such contamination, and lens cases should be replaced frequently to prevent grime and biofilm buildup.62
Antibiotics, antimicrobials: Antibiotics and antimicrobial agents may also significantly reduce normal human ocular flora levels and species. Ciprofloxacin at 0.3% has been shown to markedly reduce bacterial flora on the ocular surface within 15 min of instillation.63 In an in vitro study by Dawood and Ahmad of the antimicrobial susceptibility of ocular surface bacterial flora, isolated preoperatively from patients undergoing anterior segment intraocular surgery, 95% of coagulase-negative Staphylococcus strains were susceptible to vancomycin, amikacin and gentamicin. Between 70% and 90% were susceptible to ciprofloxacin, doxycyclin and chloramphenicol; and less than 70% were susceptible to oxacillin, penicillin, ceftriaxone, tetracycline, erythromycin and cotrimoxazole. Many factors including application frequency, concentration given, method of application (topical or subconjunctival), minimal inhibitory concentration of the drug, bioavailability, and rate of elimination from tears may impact efficacy.64
Coagulase-negative staphylococci are less pathogenic than S. aureus but are key players in line-associated bacteraemias (e.g., from intravascular catheters) and prosthetic device infections, whereupon they may form biofilms.65, 66 They are more often resistant than S. aureus to antibiotics.65 More than 80% of coagulase-negative staphylococcal isolates have been shown to be resistant to β-lactam antibiotics, semi-synthetic penicillins and methicillin.66, 67 Some hospitals have reported rates of oxacillin resistance in coagulase-negative staphylococci approaching 90%. Antibiotic resistance in coagulase-negative staphylococci, as is the case in S. aureus, is carried on a staphylococcal chromosome cassette (SCC), which almost always includes the mecA gene that conveys resistance to semi-synthetic penicillins (SCCmec).66
Additionally, these staphylococci become more resistant when growing on surfaces, which may relate to cosmetics for the prevention of biofilm formation on associated discs, films, fibers and containers in terms of spoilage during use and storage. For attached microorganisms, surface growth is slower and metabolic activity is decreased, thus the uptake of some antibiotics is blocked. The extracellular polymeric substances (including polysaccharide slime, proteins, glycolipids and extracellular DNA) produced in coagulase-negative staphylococcal biofilms and may also impact the penetration of or neutralize the activity of antibiotics, such as vancomycin.68
Alternative antibacterials: Alternative agents for the control of coagulase-negative staphylococci have been under investigation. Oguz et al. evaluated the effectiveness of the topical agent taurolidine in eradicating or reducing microorganisms in the normal flora of human eyes in a randomized, controlled study of 110 patients. S. epidermidis was the most common microorganism isolated from subjects’ eyes before therapy and its concentration was significantly reduced in taurolidine-treated subjects.69
Albietz and Lenton evaluated the effect of an antibacterial medical-grade honeyb applied topically on the ocular flora of patients with dry eyes caused by tear deficiency and/or meibomian gland disease.70, 71 The commercial blend of honey tested, including Australian manuka and New Zealand Leptospermum myrtle species, has been shown to have antibacterial properties, which like other honey, is due to its high osmolarity. Honey may also contain phytochemical components such as polyphenols and antioxidants, and can generate hydrogen peroxide via glucose oxidation when it comes into contact with sodium. The antibacterial activity of honey varies with its origin and processing.71
Results from the Albietz and Lenton study showed the microorganism colony forming units (CFUs) isolated from each of the dry eye subgroups before antibacterial honey use were significantly greater than those isolated from the non-dry eye control group. The antibacterial honey significantly reduced the total CFUs for the eyelids and conjunctiva of dry eye subjects after one month of treatment.70
As knowledge of the microbial ocular flora and its role on the ocular surface expands, implications for both ocular surface health and surface disorders continue to emerge. Recent findings suggest that ocular flora is involved in a number of functions ranging from priming surface epithelial immune cells to production and regulation of mucin composition. It is believed that it is the initial cross talk between the ocular flora and ocular surface epithelium cells that can ultimately lead to beneficial or detrimental outcomes. The differentiation relies on innate immunity receptors, e.g., transmembrane toll-like receptors, which are essential for pathogen recognition. Under normal conditions, this cross talk helps to maintain ocular surface homeostasis and health via inhibition of apoptosis, acceleration of wound repair, exclusion of pathogens and barrier preservation. The ocular ecosystem remains relatively stable throughout life, unless interrupted by antibiotics, preservatives, surgery, contact lens wear, immunocompromization or infection.72
Formulator Considerations
Considering that human flora varies from site to site on the body, the area to which a cosmetic or personal care product will be applied—in this case, the ocular area—is of prime importance when formulating a product. Preservative systems must be effective against ocular flora transmitted from consumer handling and product application as well as against environmental agents. Products targeted to multiple site applications, such as dual eye and lip creams, must be effective against flora from both areas with respect to product spoilage from these contaminants. Exposure to bacteria with developed efflux pumps and other resistance mechanisms or degradative enzymes for a particular antimicrobial agent should be of consideration and while broad-spectrum protection is warranted, perhaps preservatives may be more tailored to match the unique insult challenges for eye area-specific products.
Sustaining the balance between protecting a cosmetic product from microbial contamination and minimizing the product’s potential for preservative irritancy or other negative effects on the consumer is essential to the art of formulation.73 Awareness of the normal and transient ocular flora is helpful in the development of ocular area cosmetics so they may be adequately preserved against the often daily exposure to these microorganisms through routine use.74, 75 The cosmetic product itself may also impact the ocular flora at the point of application and knowledge of this potential is useful as well.
It has been estimated that approximately 70% of women wear eye area cosmetics. The National Purchase Diary Group Inc. (NPD), a market research group, recently reported the average age that a female begins to use beauty products is 13.7. This is much lower than the 2005 NPD reported average starting age of 17. More males have also begun using cosmetics for the eyes.76 Eye area cosmetics may be applied to the eyelashes, the ocular surface, surrounding tissues and eye lids. Recent products have been designed to be more resistant to fading and smudging, which can make their removal more difficult. Waterproof cosmetics may contain oils, waxes or silicone-based compounds that combat the effects of tears and perspiration76 and an oil-based cleanser may be required for their removal.77 Mascaras and eyeliners contain a range of pigments, oils, waxes and preservatives, and the effect of these substances on the tear film and associated dry eye/discomfort symptoms, the cornea and the ocular surface is not well-characterized.76
A recent study by Goto et al. of users who applied a hydroxyethyl cellulose gel cosmetic close to or onto the eyelash line showed migration of large amounts of product onto the ocular surface just 30 min post-application.76, 78 The migration of product increased with time and was exacerbated by saline eye drop instillation.78 Eye care practitioners have also previously reported makeup particles such as glitter being suspended in the pre-corneal tear film of patients upon slit lamp examination. In addition, the upper eye lid margin of patients has been found to be contaminated by eye makeup applied to the lower eye lid margin.76
Further, whether contact lens wearers apply makeup after their contact lenses are inserted or they remove their makeup before taking out their contact lenses, cosmetic products, especially those containing oil and silicone, may be transferred to the contact lens and, subsequently, to the eye.77 Though the vast majority of eye area cosmetics produced today are considered to be safe, the ocular migration and transfer potential of these products should be considered, especially when formulating with novel ingredients.
In relation, a study by McConville and Anderson showed that microflora of eye makeup users fluctuated from week to week in terms of both the number of microorganisms recovered and the type.74, 75 Isolates were primarily S. epidermidis and diphtheroids, with occasional M. flavus, Bacillus species, P. aeruginosa and S. aureus, in concurrence with other studies with non-makeup users.75, 79 The study was performed in Los Angeles, which is temperate and dry, and yeast and mold recovery was minimal. A study on eye flora conducted in Atlanta, however, showed higher recovery of yeasts and fungi, illustrating the effect of geological location.75
Makeup use habits and regimens have also been shown to influence the composition of the ocular flora.74, 75 One subject in the McConville and Anderson study reported using a facial cleanser containing 1.5% salicylic acid and 2.0% sulfur every day before applying makeup. This subject was the only one to show consistent recovery of S. epidermidis on all four weekly samplings. An individual having dry skin or one who frequently and fastidiously cleans the facial area would be expected to maintain a lower microbial population than an individual with oily skin utilizing a less rigorous cleaning regimen.75
A study by Wilson found that the outer eyes of women who used eye area cosmetics more often harbored pseudomonads that were subsequently transferred back to their eyeliners, mascaras and eye shadows. If the products were not adequately preserved, the microorganisms may have multiplied, and potentially infectious products may have been reapplied to the eyes.74, 80
Furthermore, when mascara and contact lenses are worn at the same time, the bacterial flora around the eye may increase, underscoring the need for adequate control of bacterial growth in the mascara tube. The personal hygiene of the user has a great impact on the preservation of mascaras. A recent study suggests that both contact lens wearers and non-contact lens wearers should replace their mascara after three months; however, many users will not replace their mascara until the tube is empty. In fact, some patients report adding water or saliva to the product to make it last longer,76 which further increases the risk of contamination.
Hygiene may also influence the development of postoperative eye infections and blepharitis.81, 82 Personal care products have been used to reduce bacterial loads on eyelids.81 A common recommendation by eye care practitioners for eye makeup removal is to dilute baby shampoo with water (1:10, or a 25% solution) and to use this dilution to moisten a sterile pad or cotton tip swab and scrub the eyelid and lid margin. Similarly, other saturated pads have been commercialized and marketed for eyelid hygiene or cleansing.81, 82 Such pads must have enough antibacterial efficacy against eyelid bacteria within clinically relevant exposure times but without causing irritation to effectively serve this purpose.81
Finally, the fingers often and effectively transfer flora from other body sites to the eye. McConville and Anderson reported a case wherein the subject’s ear infection was the source of the Staphylococcus population of her orbital area. The bacterium was likely transferred by the patient rubbing her eyes after touching the ear area with her finger. S. aureus was recovered from the subject’s eye shadow immediately after its application. However, 48 hr later, the microorganism could not be recovered from the product, indicating the robustness of the eye shadow preservative.83 This illustrates the benefit of knowing the flora in terms of protection against microorganisms likely to be introduced into product by direct application.75
Conclusion: Parts 1 and 2
The resident and transient flora of the human skin and the ocular area are comprised of a varied mixture of microorganisms. The composition of the flora is influenced by a number of factors including age, immune state, environment and the presence of antimicrobial agents. This diversity should be considered as cosmetic and personal care formulation preservative systems, antimicrobial agents, chemical composition, target age and points of use are selected and developed. As the knowledge of human flora expands, new cosmetic applications may arise for the control of pathogenic skin and ocular area microorganisms, or the nourishment of advantageous ones.
References
Editor’s note: The following references are a continuation from the first of this two-part article, and are numbered accordingly.
39. GAJ Ayliffe, The effect of antibacterial agents on the flora of the skin, J of Hospital Infection 1 111–124 (1980)
40. S Selwyn, Microbiology and ecology of the human skin, The Practitioner 224 1059–1062 (Oct 1980)
41. N Hua et al, Normal conjunctival flora in healthy infants aged from 1 to 4 months, Zhonghua Yan Ke Za Zhi, Tianjin Medical University Eye Center, Tianjin, China 46(6) 537–541 (Jun 2010)
42. JA Capriotti et al, Normal ocular flora in healthy eyes from a rural population in Sierra Leone, Int Ophthalmol 29(2) 81–84 ( Apr 2009)
43. J Pelletier and J Capriotti, Take a closer look at the ocular flora, Ophthalmology Management, available at http://www.ophthalmologymanagement. com/articleViewer.aspx?articleID=103819 (Accessed Jan 31, 2012)
44. J Cabrera and J Rodriguez, Ocular bacterial flora in contact lens wearers, ICLC 23 149–151 (Jul/Aug 1996)
45. RP Kowalski and MI Roat, Normal flora of the human conjunctiva and eyelid, ch 41, Duane’s Ophthalmology Vol 2, Lippincott, Williams and Wilkins: Philadelphia, USA, available at www.oculist.net/downaton502/prof/ebook/duanes/pages/v8/v8c041.html (Accessed Jan 31, 2012)
46. EC Alfonso et al, Fungal infections, and G Smolin and RA Thoft, Part II, Clinical topics, bacteriology, in Smolin and Thoft’s The Cornea: Scientific Foundations and Clinical Practice, CS Foster, DT Azar and DH Dohlman, eds, Lippincott, Williams & Wilkins: Philadelphia, USA (2005)
47. PE Dantas et al, Microbiological study of disposable soft contact lenses after photorefractive keratectomy, CLAO J 26 26–29 (Jan 2000)
48. Q Dong et al, Diversity of bacteria at healthy human conjunctiva, Invest Ophthalmol Vis Sci 52 8 5408–5413 (Jul 20, 2011)
49. M Eder et al, Normal ocular flora in newborns delivered in two hospital centers in Argentina and Paraguay, Graefe’s Arch Clin Exp Ophthalmol 243 1098–1107 (2005)
50. S Isenberg et al, Source of the conjunctival bacterial flora at birth and implications for ophthalmia neonatorum prophylaxis, Amer J Ophthalmol 106 458–462 (1988)
51. H Thiel and U Schumacher, Normal flora of the human conjunctiva; Examination of 135 persons of various ages, Klin Monatsbl Augenheilkd 205 348–357 (1994)
52. RS Lowery, Adult blepharitis, MedscapeReference Drugs, Diseases and Procedures, available at https://emedicine.medscape.com/article/1211763-overview (Accessed Jan 31, 2012)
53. CS Foster, Dry eye syndrome, MedscapeReference Drugs, Diseases and Procedures, available at https://emedicine.medscape.com/article/1210417-overview (Accessed Jann 31, 2012)
54. VPS Tomas et al, Bacterial and fungal flora of normal conjunctiva, Ann Ophthalmol 3 669 (1971)
55. N Sharma et al, Fungal keratitis, in Textbook of Ophthalmology, Vol 2, S Agarwal et al, eds, Jaypee Brothers Medical Publishers: New Delhi, India (2002)
56. A al-Hemidan et al, Bacillus cereus panophthalmitis associated with intraocular gas bubble, Br J Ophthalmol 73 25–28 doi:10.1136/bjo.73.1.25 (1989)
57. OP van Bijsterveld and RD Richards, Bacillus infections of the cornea, Arch Ophthalmol 74(1) 91–95 (1965)
58. WL Mackenzie, Methods of disinfection in Public Health Vol 12, 599, Society of Community Medicine, Society of Medical Officers of Health, Incorporated Society of Medical Officers Health: Boston, USA (1900)
59. D Locatcher-Khorazo and BC Seegal, Microbiology of the eye, CV Mosby Company: St. Louis, USA (1972)
60. MC Pramhus et al, Ocular flora in the severely burned patient, Arch Ophthalmol 96(8) 1421–1424 (1978)
61. I Alarcon et al, Role of the corneal epithelial basement membrane in ocular defense against Pseudomonas aeruginosa, Infection and Immunity 3264–3271 (Aug 2009)
62. CF Amos and MD George, Clinical and laboratory testing of a silver-impregnated lens case, Contact Lens and Interior Eye 29 247–255 (2006)
63. L Snyder-Perlmutter et al, Effect of topical ciprofloxacin 0.3% and ofloxacin 0.3% on the reduction of bacterial flora on the human conjunctiva, J Cataract & Refractive Surgery 26 11 1620–1625 (2000)
64. A Dawood and J Ahmad, Antibiotic resistance patterns of ocular surface bacterial flora, Infect Dis Antimicrob Agents 22 2 (May–Aug 2005)
65. DM Livermore, Antibiotic resistance in staphylococci, Interl J Antimicrobial Agents Sup 1 S30–S10 (Nov 16, 2000)
66. JF John and AM Harvin, History and evolution of antibiotic resistance in coagulase-negative staphylococci: Susceptibility profiles of new anti-staphylococcal agents, Therapeutics and Clinical Risk Management 3(6) 1143–1152 (Dec 2007)
67. JM Tufariello et al, Coagulase-negative staphylococci: Antimicrobial resistance and treatment, available at www.uptodate.com/contents/coagulase-negative-staphylococciantimicrobial-resistance-and-treatment (Accessed Jan 31, 2012)
68. RA Proctor, Editorial response: Coagulase- negative staphylococcal infections: A diagnostic and therapeutic challenge, Clinical Infectious Diseases 31 1 31–33 (2000)
69. H Oguz et al, Effect of taurolidine on the normal eyelid and conjunctival flora, Current Eye Research 21 5 851–855 (2000)
70. JM Albietz and LM Lenton, Effect of antibacterial honey on the ocular flora in tear deficiency and meibomian gland disease, Cornea 25(9) 1012–1019 (Oct 2006)
71. RA Cooper et al, Antibacterial activity of honey against strains of Staphylococcus aureus from infected wounds, J Royal Society of Medicine 92 283–285 (Jun 1999)
72. D Miller and A Iovieno, The role of microbial flora on the ocular surface, Current Opinion in Allergy and Clinical Immunology 9 466–470 (2009)
73. B Katusin-Razem et al, Microbial decontamination of cosmetic raw materials and personal care products by irradiation, Radiation Physics and Chemistry 66 309–316 (2003)
74. FN Marzulli et al, Induced Pseudomonas keratitis as related to cosmetics, J Soc Cosmet Chem 23 89–97 (1972)
75. JF McConville and DW Anderson Jr, Aerobic microflora of the outer eye area of women of Los Angeles, J Soc Cosmet Chem 26 83–91 (1975)
76. C Hudson, Impact of cosmetics on the ocular surface and contact lens wear, Optometry Continuing Education and Training Course Code C-16942/O/D/CL, available at www.optometry.co.uk/uploads/exams/articles/cet_30_sept_2011_hudson.pdf (Accessed Dec 2, 2011)
77. CW Sindt, Can cosmetics impact contact lens wear? Review of Cornea & Contact Lenses, www.reviewofcontactlenses.com/content/c/28735 (Accessed Dec 2, 2011)
78. T Goto et al, Cosmetic product migration onto the ocular surface: Exacerbation of migration after eye drop instillation, Cornea 29 (4) 400–403 (2010)
79. Amaechi and PC Inyanga, A comparative study of the conjunctival bacterial normal flora in paediatric and geriatric subjects, JNOA 16 20–22 (2010)
80. LA Wilson et al, Microbial contamination in ocular cosmetics, Amer J Ophthalmol 71 1298 (1971)
81. US Patent Application 20070243275, Methods and Compositions for the Treatment of Infection or Infectious Colonization of the Eyelid, Ocular Surface, Skin or Ear, JP Gilbard, Weston, MA (Oct 18, 2007)
82. Y Miranda and Y Antonieta, Efficacy of 0.5% Levofloxacin therapy against anaerobic bacterial flora in chronic-blepharoconjunctivitis patients: A prospective semi-randomized study, poster presentation at The Association for Research in Vision and Ophthalmology (ARVO) Annual Meeting, Fort Lauderdale, FL, USA (May 2008)
83. JF McConville and DW Anderson Jr, Occurrence of Pseudomonas aeruginosa and Staphylococcus aureus in the orbital area, J Soc Cosmet Chem 26 169–172 (1975)