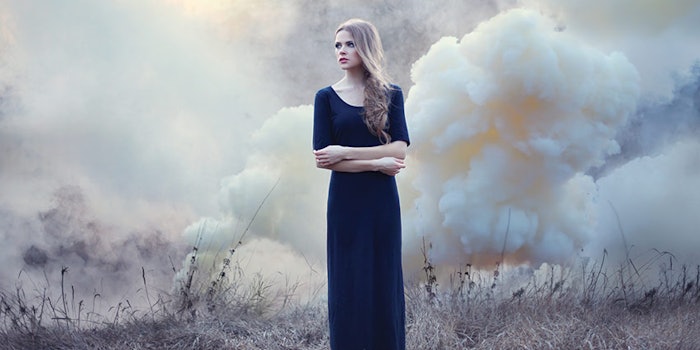
Air pollution is one of the main environmental risks to health. The World Health Organization estimates roughly seven million premature deaths per year are directly related to air pollution.1 While many of these fatalities were attributed to conditions including acute lower respiratory disease, chronic obstructive pulmonary disease and ischaemic heart disease, one would be remiss to dismiss the particulates in air pollution whose components settle on the skin and cause damaging effects.
Indeed, it has been shown that the smallest particulate matter (PM) can enter and accumulate in the hair follicles up to 2 mm deep,2 promoting the contact of keratinocytes and melanocytes with metals and organic pollutants associated with PM. Moreover, Fiorito et al.3 showed that the particulate matter from diesel engines (DPMs) were ingested spontaneously by keratinocytes in culture.
DPMs are loaded with oxidant, irritant, mutagen and carcinogen molecules such as benzo[a]pyrene (B[a]P). Also present in cigarette smoke, B[a]P easily penetrates into skin cells where it is transformed into highly toxic by-products by cellular enzymes. B[a]P associates with the protein complex called the aryl hydrocarbon receptor (AhR), which translocates into the nucleus and highly upregulates the CYP1 family of genes. These Phase-I CYP1 enzymes unfortunately convert B[a]P into toxic and mutagenic by-products and create harmful ROS, which breaks cellular homeostasis and causes lipid peroxidation, DNA damage, protein carbonylation and the production of proinflammatory cytokines such as IL-8.4-6
To defend against these events, keratinocytes have an innate cytoprotective and decontaminating mechanism using Phase-II enzymes and connected to the Nuclear factor erythroid 2-Related Factor 2 (NRF2). Interestingly, some phytochemicals such as forsythoside B can combine with NRF2 to generate the formation of detoxifying enzymes, e.g., NQO1, HMOX, GPXs, etc., which can then inhibit the deleterious effect of pollutants.6-8
In addition to DPMs, ozone (O3) is a highly irritating gas produced by UV radiation and human activities, which induces inflammation pathways through the production of cytokines. In addition, it generates the oxidation of stratum corneum molecules; produces malondialdehyde (MDA); destroys the natural pool of vitamins E and C; and produces DNA damage.9-11
Thus, in relation to skin, air pollutants induce direct or indirect changes and the metabolic dysfunctioning of skin components, leading to increased cell mortality.12 This could explain the results of epidemiological studies performed in European and Chinese populations showing that pollution is correlated with skin defects and signs of premature skin aging, such as lines, wrinkles and age spots.13, 14 In addition, Lefebvre et al.15 have shown that, for individuals living in the highly polluted environment of Mexico, the quality of their epidermis was modified, presenting with a weakened skin barrier, less ATP and more oxidized proteins; moreover, skin was significantly oilier and less hydrated. These effects lead to increased skin aging, inflammation or allergies favoring the development of atopic dermatitis, psoriasis, acne and cancer.12, 16 It also seems clear that the phenomenon of sensitive skin, associated with a dysfunction of the skin barrier, is much more prevalent in highly urbanized areas.
In consideration of these damaging pathways, a preliminary screening of plant extracts was conducted to test for lipid, protein and cell protection. From this, Marrubium vulgare extract, an edible plant used in Roman traditional medicine and containing forsythoside B, was selected and produced by a plant cell culture technology. As described next, tests were performed using an optimized extracta to determine whether it could stimulate anti- and de-pollution pathways, e.g., NRF2, glutathione and autophagy, in skin cells to reinforce epidermis defense and skin barrier functions against environmental pollutants.
Test Materials
Extract preparation: Marrubium vulgare cell cultures, obtained by an eco-friendly biotech incubation process, were collected and prepared in a manner to ensure a cell suspension with a constant concentration of forsythoside B (350 ppm)b. For in vitro tests, cells were collected on a sterile 50 μm Buchner filter and lyophilized prior their extraction into cold ethanol using an ultrasound system. Solutions of Marrubium vulgare extract (MVE) were tested on keratinocytes at a rate of 1.75-7.0 ppm of forsythoside B; the solvent was 0.1% ethanol. For analytical purposes, purified forsythoside B molecules were obtained from IRB as described in Reference 8.
Particulate matter: DPMs were provided by the USA and suspensions were prepared in cell culture media. B[a]P was obtainedc and test solutions were prepared into dimethyl sulfoxide (DMSO). For test purposes, keratinocytes received either 0.1% ethanol or 0.1% DMSO as solvent, or both together.
Keratinocytes and media: Dulbecco's modified Eagle's medium (DMEM); keratinocyte serum-free medium (K-SFM); human recombinant epidermal growth factor (EGF); bovine pituitary extract (EBP); fetal calf serum (FCS); and penicillin-streptomycin (10,000 IU/ L penicillin and 10 mg/mL streptomycin) all were suppliedd.
Human keratinocyte (HK) cultures were incubated either in K-SFM supplemented with penicillin, streptomycin, EGF and EBP, or in DMEM supplemented with 3-10% FCS, penicillin, streptomycin and L-glutamine. For daily maintenance, cells were seeded in 175-cm2 flask in their culture medium and incubated at 37°C in a humidified 5% CO2 atmosphere. Media were renewed every 48-72 hr, to allow for outgrowth. For all subsequent experiments, keratinocytes were trypsinized and seeded in multi-well (MW12, 24 or 96) plates in their culture medium.
In vitro Tests
Once subconfluence was reached, cells were treated for 1-72 hr with MVE, with or without pollutants—i.e., DPM, B[a]P or O3, in a specific chambere. Then, cells quantification or viability tests were performed either using nuclei labeling with propidium iodide/Hoechst or using MTT/Uptiblue assay. For the assessment of the following endpoints, cell extracts, cell supernatants, ribonucleic acids or cell monolayers were used.
To evaluate DPM internalization into HK, the following were assessed: DPM-induced upregulation of CYP1A1 mRNA; DPM-induced AhR translocation; and DPM-induced intracellular ROS; here, DPMs of 0.3-300 μg/mL were used for 3 hr-72 days. Intracellular ROS induced in HK either by DPMs or O3 were quantified using the method of Rosenkranz et al.,17 with minor modifications. Protein carbonyl groups and lipid peroxidation, both DPM-induced in HK, were evaluated by a third partye.
IL-8 synthesis was quantified in HK supernatants after contact with MVE, with or without B[a]P (0.3-30 μM) or DPMs using the ELISA methodf; results were normalized as described previously.18
Autophagy vacuoles quantification was performed in HK after contact with MVE, with or without DPM; labeling was achieved using an autophagy detection kitg. Fluorescence was recorded under a microscopeh and analyzed using softwarej.
For AhR translocation studies, protein fractions were prepared for a Western Blot evaluation and transferred onto a nitrocellulose membrane. AhR was tagged using a specific antibody bearing chemiluminescence probe. For quantification, images of the membrane were captured using a charge-coupled device (CCD) camerak.
Glyceraldehyde 3-phosphate dehydrogenase (GAPDH) was used as a reference protein to normalize the data.19
After contact with B[a]P, DNA damage was assessed in HK using a Comet assaym according to the manufacturer’s instructions. DNA was stained by SYBR Green I and comets were analyzed under a fluorescence microscopeh and with softwaren. The percentage of fluorescence in the tail versus the entire comet was used to quantify the signals.
Autophagy is reduced with age but even more so in the presence of diesel particulate matter, which has been shown to decrease the autophagy activity of white blood cells.
For RNA extraction and quantitative Reverse Transcription-Polymerization Chain Reaction (qRT-PCR) studies, HK RNA was extracted using a kitp according to manufacturer’s instructions. The reverse transcription assay was carried out using another kitq and 0.8-1 μg of total RNA. Following, qRT-PCR products were generated from 50 ng of cDNA template using a specified reagentr with specific forward and reverse primers for each gene of interest provided by the manufacturerr. β2-microglobulin or GAPDH served as housekeeping genes. The two steps of qRT-PCR were performed with a thermal cyclers. For DNA-array studies, total RNAs were extracted as described earlier from HK treated with or without MVE and DPM. The mRNAs were then converted to cDNA, then DNA-array analyses were conducted on chipst.
Skin Equivalent Tests
Human Living Skin Equivalents (LSE) were produced in-house following the method of Asselineau et al.,20 with modifications. Normal human fibroblasts (NHF) were seeded into collagen-I matrix and maintained in a specific medium to obtain a reconstructed dermis. HK were then seeded onto the matrix, where they migrated and multiplied for a few days. During the immersion step, HK differentiated and provided an epidermis. Seven days later, the LSE received MVE and solvent in the culture medium. Following this, a thin film of culture medium containing DPM was spotted onto the LSE surface in order to avoid complete stratum corneum damage.
After three days of contact, LSEs were cryofixed using liquid nitrogen and a specified compoundu, sectionedv and stainedw. Their thicknesses were quantified under a microscopex using softwarey and results were compared versus solvent as the negative control.
All experiments were performed minimally in triplicate, if not in pentaplicate, and the mean value of replicates in each comparable group was analyzed using the student's t-test.
Results: DPM Internalization
Results of the present work confirmed the Fiorito observations3—that DPMs can be ingested by living HK. This study showed they were localized around the nucleus of HKs (see Figure 1). MVE (500 ppm), however, greatly limited the internalization of DPMs; analysis of the images captured indicated a 76% reduction (p < 0.01 vs solvent). Furthermore, no cell toxicity was observed.
Results: CYP1A1 Gene Expression and AhR Translocation
As described, CYP1A1 mRNA regulation in HK was evaluated after a 24-hr contact period with or without MVE. As expected, qRT-PCR studies showed that DPMs upregulated CYP1A1 mRNA by 19.6× (p < 0.01); MVE, however, reduced this upregulation by 47%; i.e., registering only a 10.0x upregulation; p < 0.01 (data not shown).
For AhR translocation tests, HKs were first contacted with 500 ppm of MVE for 1 hr, then with DPMs for 3 hr. After rinsing, Western Blot revealed that translocation of AhR in the nucleus was greatly increased with DPM application alone; the ratio of AhR in the nucleus versus cytoplasm increased from 1 to 3.7, or 266%. With MVE, however, this translocation was significantly reduced by 82% (data not shown).
These results revealed that MVE could limit the internalization of DPMs, down-regulate CYP1A1 mRNA, and reduce the translocation of AhR. These effects suggest the extract limited the harmful effects of DPMs and reduced the activation of stress mechanisms.
Results: Intracellular ROS, Carbonyl Proteins and Lipid Peroxidation
As expected, keratinocytes stressed with either DPMs or O3 showed a strong increase in intracellular ROS production; 233% and 155%, respectively (both p < 0.01). When treated with 287-500 ppm of MVE, though, intracellular ROS were reduced by 74% (p < 0.01) after DPM exposure, and by 52% (p < 0.01) after O3 (data not shown).
DPMs applied to keratinocytes also induced a strong 50% increase in carbonylated proteins vs the negative control (p < 0.01). However, 500 ppm of MVE reduced this production by 26% (p < 0.01), resulting in just a 10.8% increase in carbonylated proteins vs the negative control (data not shown).
A third series of experiments was performed to evaluate the lipid peroxidation of DPMs in cells and the potentially protective effects of MVE (500 ppm). Lipid peroxidation was assessed by the presence of malonaldehyde (MDA), a mutagenic by-product. Results showed that DPMs significantly increased MDA amounts by 118% versus negative control (p < 0.01); in parallel, MVE reduced DPMs-induced MDA levels by 38.9% (p < 0.01) (data not shown).
Results: Pollutant-induced DNA Damage
The comet assay21-23 used to highlight DNA damage induced in HK by B[a]P indicated this highly penetrating pollutant, vectored by DPMs, causes a strong increase in DNA breaks (+210% vs negative control). These are shown in Figure 2a (arrows); 625 ppm of MVE, in contrast, significantly protected cells against DNA damage; i.e., a 21% reduction in comets vs B[a]P alone (p < 0.01; see Figure 2b).
Results suggest the Marrubium vulgare extract limited the harmful effects of diesel particulate matter and reduced the activation of stress mechanisms.
Results: Pollutant-induced IL-8 Release by HK
HKs contacted by DPM or B[a]P triggered an increase in IL-8 release by 70% and 49.5%, respectively (both p < 0.01). Application of MVE (625 ppm), significantly reduced IL-8 production by 67% and 79%, respectively (both p < 0.01). Thus, MVE reduced this pre-inflammatory mediator at its origin; i.e., at the point where it would recruit pro-inflammatory cells and amplify the inflammation process. Indeed, MVE reduced IL-1α induced by B[a]P by 32% (p < 0.01) (data not shown).
Results: DPMs-induced Autophagy Reduction
Autophagy, i.e., the protective mechanism of cells, is reduced with age;24 but even more so in the presence of DPMs, which have been shown to decrease the autophagy activity of white blood cells.25 Based on these observations, the effects of DPMs on autophagy were also tested as described above, using autophagy vacuoles quantification. Results indicated DPMs decreased this mechanism in HK by 51% (p < 0.01)—but MVE strongly reduced this effect by 64% (p < 0.01). As such, it was concluded MVE could counteract the effects of pollution on skin’s autophagy mechanism (data not shown).
Results: DNA Array of Cytoprotective Genes
Analysis of the DNA-array performed on HK showed that in the presence of DPMs, MVE stimulated the NRF2 protection pathway of the cells. MAFG mRNA, in particular, was over-expressed in response to MVE, and it is known that once this protein is associated to NRF2, it can promote the expression of various genes involved in defense, detoxification and cytoprotection such as NQO1, HMOX-1, GPX2, GPX3, SLC7A11, GLRX and ALDH3A1—all of which are induced by MEV (data not shown).
Results: Skin Equivalent Tests
As stated, LSEs were exposed to DPMs applied to their stratum corneum for two days. Interestingly, a clear change in the compactness of the stratum corneum was observed, as well as a significant 29% decrease in the total epidermis thickness (p < 0.01). In parallel, MVE preserved the compactness of the stratum corneum and reduced the deleterious effects of DPMs on the thickness of the epidermis to just 18.6% (p < 0.01 vs DPMs alone; see Figure 3).
Results: DNA Array of Natural Cutaneous Defenses
In addition to the previous qRT-PCR study, it was shown that MVE (500 ppm) significantly upregulated the HK mRNAs of involucrin by 15x versus negative control; transglutaminase-1 by 3x versus negative control; and filaggrin by 5.2x versus negative control. The DNA array also confirm that MVE: upregulated the LCE3A-E, cornulin (CRNN), tight junction (TJP3) and small proline rich protein (SPRR-2G and -4, KPRP) mRNAs (data not shown).
Conclusions
The present work confirmed and demonstrated that pollutants can act on skin cells, and on living skin equivalents (LSE) at gene and protein levels. Pollutants triggered various stress signals, causing negative effects to cells such as DNA damage, lipid peroxidation, protein carbonylation and cytokine release. In addition, it was shown that DPMs are internalized by keratinocytes and localized around the nucleus where components carried by DPMs could be released close to DNA. Further, LSE assays showed that DPMs reduced the thickness of epidermis and caused clear modifications in its compactness.
The Marrubium vulgare extract (MVE) of interest, however, was shown to stimulate defense mechanisms and improve detoxification mechanisms in skin cells, in turn reinforcing the skin barrier and more generally, fighting against the damaging effects of pollutants. Furthermore, initial results obtained from clinical evaluations (not shown) indicate that MVE could provide an effective anti-aging strategy related to city aerial pollution.
References
- World Health Organization (WHO) (2014). Burden of Disease from Household Air Pollution for 2012. Retrieved from https://www.who.int/phe/health_topics/outdoorair/databases/FINAL_HAP_AAP_BoD_24March2014.pdf.
- Lademann, J., Schaefer, H., Otberg, N., Teichmann, A., Blume-Peytavi, U., and Sterry, W. (2004). Penetration of microparticles into human skin. Hautarzt, 55, 1117-1119.
- Fiorito, S., Mastrofrancesco, A., … Picardo, M., et al. (2011). Effects of carbonaceous nanoparticles from low-emission and older diesel engines on human skin cells. Carbon, 49, 5038-5048.
- Choi, H., Shin, D. W., Kim, W., Doh, S. J., Lee, S. H., and Noh, M. (2011). Asian dust storm particles induce a broad toxicological transcription program in human epidermal keratinocytes. Toxicol Lett, 200, 92-99.
- Costa, C., Catania, S., De Pasquale, R., Stancanelli, R., Scribano, G. M., and Melchini, A. (2010). Exposure of human skin to benzo[a]pyrene: Role of CYP1A1 and aryl hydrocarbon receptor in oxidative stress generation. Toxicology, 271, 83-86.
- Furue, M., Takahara, M., Nakahara, T., and Uchi, H. (2014). Role of AhR/ARNT system in skin homeostasis. Arch Dermatol Res, 306, 769-779.
- Natarajan, V. T., Singh, A., … Gokhale, R. S., et al. (2010). Transcriptional upregulation of Nrf2 dependent phase II detoxification genes in the involved epidermis of vitiligo vulgaris. J Invest Dermatol, 130, 2781-2789.
- Sgarbossa, A., Dal Bosco, M., Pressi, G., Cuzzocrea, S., Dal Toso, R., and Menegazzi, M. (2012) Phenylpropanoid glycosides from plant cell cultures induce heme oxygenase 1 gene expression in a human keratinocyte cell line by affecting the balance of NRF2 and BACH1 transcription factors. Chem-Biol Inter, 199, 87-95.
- Thiele, J. J ., Traber, M. G., Tsang, K., Cross, C. E., and Packer, L. (1997a). In vivo exposure to ozone depletes vitamins C and E and induces lipid peroxidation in epidermal layers of murine skin. Free Radic Biol Med, 23, 385-391.
- Thiele, J. J ., Podda, M., and Packer, L. (1997b). Tropospheric ozone: An emerging environmental stress to skin. Biol Chem, 378, 1299-1305.
- Weber, S. U., Thiele, J. J., Cross, C. E., and Paker, L. (1999). Vitamin C, uric acid and glutathione gradients in murine stratum corneum and their susceptibility to ozone exposure. J Invest Dermatol, 113, 1128-1132.
- Drakaki, E., Dessinioti, C., and Antoniuo, V. (2014). Air pollution and the skin. Frontiers in Environ Sci, 2, 1-6.
- Li, M., Vierkötter, A., … Wang, S., et al. (2015) Epidemiological evidence that indoor air pollution from cooking with sold fuels accelerates skin aging in Chinese women. J Dermatol Sci, 79, 148-154.
- Vierkötter, A., Schikowski, T., Ranft, U., Sugiri, D., Matsui, M., Krämer, U., and Krutmann, J. (2010). Airborne particle exposure and extrinsic skin aging. J Invest Dermatol 130, 2719-2726.
- Lefebvre, M. A., Pham, D. M., Boussouira, B., Bernard, D., Camus, C., and Nguyen, Q. L. (2015). Evaluation of the impact of urban pollution on the quality of skin. A multicenter study in Mexico. Int J Cosmet Sci, 37, 329-338.
- Baudouin, C., Charveron, M., Tarroux, R., and Gall, Y. (2002). Environmental pollutants and skin cancer. Cell Biol Toxicol, 18, 341-348.
- Rosenkranz, A. R., Schmaldienst, S., Stuhlmeier, K. M., Chen, W., Knapp, W., and Zlabinger, J. (1992). A microplate assay for the detection of oxidative products using 2’-7’-dichlorofluorescein-diacetate. J Immun Meth 156, 39-45.
- Mondon, P., Dal Toso, R., Ringenbach, C., Lavaissière, L., Doridot, E., Ouvrat, E., and Brahimi, S. (2016). Evaluation of molecules or extracts modulating seborrhea and its consequences, using normal human culture of keratinocytes, skin explants models and in vivo methods. A case study. 28th IFSCC Congress, Paris.
- Johnson, M. (2012). Loading Controls for Western Blots. Retrieved from Labome.com/methods
- Asselineau, D., Bernhard, B., Bailly, C., and Darmon, M. (1985). Epidermal morphogenesis and induction of 67 kD keratin polypeptide by culture of human keratinocytes at the liquid-air interface. Exp Cell Res, 159, 536-539.
- Gyori, B. M., Venkatachalam, G., Thiagarajan, P. S., Hsu, D., and Clement, M. V. (2014). OpenComet: An automated tool for comet assay image analysis. Redox Biol, 9, 457-465.
- Karlsson, H. L. (2010), The comet assay in nanotoxicology research. Anal Bioanal Chem, 398, 651-666.
- Collins, A. R., Oscoz, A. A., Brunborg, G., Gaivao, I., Giovanelli, L., Kruszewski, M., Smith, C. C., and Stetina, R. (2008). The comet assay: Topical issue. Mutagenesis, 23, 143-151.
- Pierdominici, M., Maselli, A., … Fiorito, S., et al. (2014). Diesel exhaust particle exposure in vitro impacts T lymphocyte phenotype and function. Part Fibre Toxicol, 14, 74.
- Rubinsztein, D. C., Mariño, G., and Kroemer, G. (2011). Autophagy and aging. Cell, 146, 682-695.