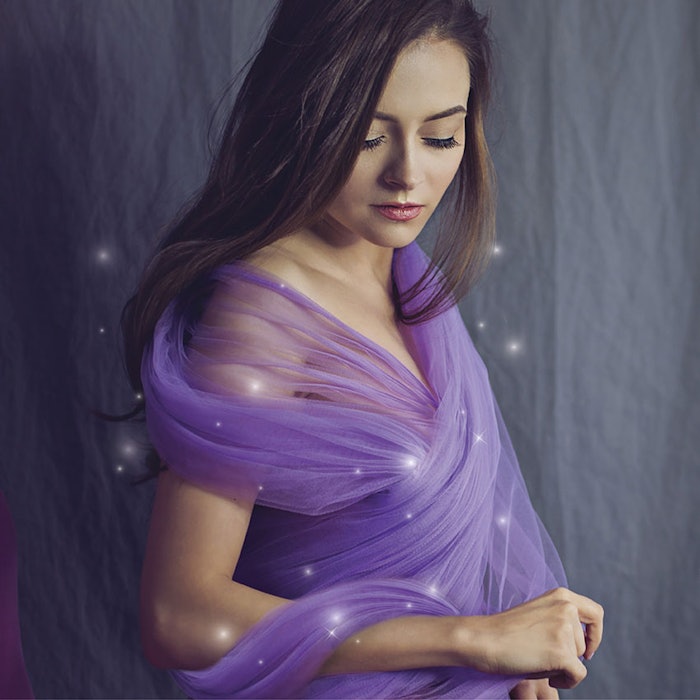
The pursuit of younger-looking skin drives cosmetic market growth. Youthful, glowing skin is achieved by advanced formulations and their accurate delivery to defined areas of the skin; typically via creams, gels or serums. Too often, the active formulation stays on the stratum corneum layer, where it delivers only minimal and temporary results. Thus, for acute delivery and deeper penetration of actives, penetration enhancers and delivery systems have been developed both by biomedical and cosmetic researchers.1
In relation, nanomaterials have been employed to both protect active ingredients and control their delivery so they can work within skin to improve its appearance. As an alternative to traditional nanoemulsions and nanoliposomes, a novel nanotechnology was developed to encapsulate and deliver bioactive agents into the skin layers quickly, for heightened benefits. Here, the authors described its manufacture and put it to the test.
The technologya comprises marine collagen nanofibers (MCN)2 that can incorporate various bioactives, such as the hyaluronic acid (HA)b and polyphenolic compoundsc utilized here. The commercially available hyaluronic acid was chosen for both its known cosmetic benefits as well as challenging characteristics for encapsulation; i.e., molecular complexity, hydrophilicity and tendency to separate out during formulation.
Collagen is used widely in medicine and oral health supplements, usually in the forms of small peptides or gelatins, to enhance extracellular matrix construction (ECM) and the synthesis of essential nutrients for skin, hair and nails.3 The collagen protein has a high substantivity to the skin3, 4 but cannot permeate due to its bulk structure. Therefore, in topical formulations, its use is limited to moisturizers or natural humectants;3, 5 or, in cosmetic surgery, it is directly injected into skin to treat minor imperfections and wrinkles.
The main objective of the present work was to construct and use collagen as a carrier and delivery vehicle for actives. The authors therefore fabricated a specialized marine collagen—i.e., disentangled α-chains of high molecular weight (HMW), denatured whole chain collagen (DWCC), with active bonding sites—in a nanofiber form using electrospinning. The combination of this unique DWCC format and electrospinning technique resulted in a highly functional material with increased surface area and superior skin penetration efficiency.
Furthermore, the fabricated MCN matrix is found to break up rapidly into small, dissolvable pieces due to its high surface area in water, deploying a strategic release of actives. The working mechanism of MCN is explained in detail and put to the test in the forthcoming sections.
Materials and Methods
Collagen sourcing: The DWC collagen was derived from the unused skins of hoki fish (Macruronus novaezelandiae) (see Figure 1a), sourced from the deepest regions of the Southern and Western oceans around New Zealand using sustainable practicesd. The fish skins are cleaned of adhering scales, fat, pigments and muscles before being subjected to the denaturalization process. The resulting collagen was used to construct MCN using a proprietary electrospinning processe.
Morphology and characterization: Scanning electron microscopy (SEM)f (see Figure 1b) and ATR-Fourier-transform infrared (FTIR) spectroscopy were usedg, respectively, to examine the morphology of fibers and to characterize the MCN samples.
Permeation: Piglet skin was chosen for penetration studies due to its physiological and anatomical similarities with human skin, specifically its permeability behavior.6 For example, the skin layers in both species have a relatively thick epidermis that is separated from all the sub-layers. The dermis of both species also contains an abundance of elastic tissue with similar collagen fibril thicknesses.7, 8
Stillborn piglet skin samples of different sizes were sourced from a pig farm, immediately processed, cut to the necessary size and preserved following a thorough tissue preservation protocol. The samples were dissected in dimensions to fit the size parameters of the imaging system's probes. On average, skin samples were 10 mm × 10 mm, with a thickness of up to 4 mm.
OCT: Optical Coherence Tomography (OCT) was usedh to assess the permeation of bioactives into skin. The system comprises a light source with a central wavelength of 1,325 nm and a bandwidth of approximately 100 nm; having a scanning rate of 16 kHz; and an output power of the probing light at 12 mW. This system is capable of acquiring 3D volumes of 1,024 pixels × 1,024 pixels × 512 pixels—i.e., up to 10 mm × 10 mm × 3 mm and containing 1,024 images—within approximately 40 sec, with axial and lateral resolutions of approx. 13 μm and 25 μm, respectively. Permeability changes can be examined up to 2.5 mm in depth.
Fundamentally, the diffusion of bioactives into a substrate changes the scattering properties, which are detectable by OCT. By utilizing specialized software, double-correlation analysis of 2D/3D OCT images are reorganized for validation of the skin penetration outcomes. OCT is a proven scientific method for non-invasive and non-destructive, functional, real-time spectral imaging. As noted, it can provide images in two and three dimensions, in vitro and in vivo.9
MCN Properties and Construction
Apart from cultural reasons and a low risk for transmitted disease,10, 11 marine collagen is preferred for its proline and hydroxyproline profile, which is high enough to deliver moisturizing effects yet low enough to electrospin. For example, the reported values of the proline plus hydroxyproline content, as a percentage of the total amino acid profile in collagen, are 14.7% for hoki skin,12 compared with 23.2% for calf skin.13
In general, the presence of proline and hydroxyproline molecules in collagen restricts the rotation of the backbone of the protein chain in all species (including humans), which supports the helix formation in the individual alpha chains of the collagen. The individual alpha chain then cross-links with two more alpha chains to form a super triple helix structure of the native collagen molecule. The hydroxyproline is also responsible for stabilizing the hydrogen bonds within the triple helix.14 This triple helix format is required to build collagen fibrils responsible for ECM construction in the body. However, it is difficult to deliver triple helix format collagen directly through the skin without rupturing its structure.
The authors believe that electrospinning an alternate form of collagen with intact telopeptides (to represent a structure closer to that of native collagen) plus proline and hydroxyproline (essential amino acids for collagen synthesis) creates perhaps the best possible natural skin delivery platform. Therefore, a specific extraction and denaturation process was carried out to produce DWCC with intact telopeptidesj.
In detail, the marine collagen is extracted from an intact triple helix (tropocollagen molecules) to produce DWCC in a weak acid solution. The presence of acid-soluble covalent cross-links specific to marine species makes it possible to recover DWCC with intact telopeptides (non-helical portions of alpha chains).12, 15 These intact telopeptides in the molecular chain make the DWCC easier to cross-link with suitable actives during electrospinning.
An intelligent delivery system will carry actives deeper into skin where they can live up to their full potential and work within skin for long-term effects.
DWCC is denatured collagen but structurally different from gelatin. Gelatin is the complex mixture of oligomers joined by covalent bonds and partially hydrolyzed, but with alpha chains having varying molecular weights.14, 16 In gelatin, the alpha chain loses its natural triple helix structure and becomes individual short peptide chains with no telopeptides at the end.16
Due to the significant structural differences between DWCC and gelatin, it was expected to electrospin in a manner different from gelatin; even with the same solvents. Interestingly, in 100% deionized water or benign acid solutions, DWCC forms a viscous liquid with solution parameters, e.g., conductivity, viscosity and surface tension, and hydrodynamic properties appropriate for electrospinning. In comparison, native atelocollagen or gelatin behaves like a gel at the concentration relevant to electrospinning. Therefore, stronger (often toxic) solvents are used to rupture the hydrogen bonding in gelatin to stretch it for electrospinning.
The literature suggests electrospinning native collagen extracted from mammalian species uses 1,1,1,3,3,3-hexafluoro-2-proposal (HFP) to disrupt the hydrogen bonding that stabilizes the helix.17, 18 However, HFP is categorized as a dangerous fluorinated solvent and therefore is not desirable for electrospinning collagen nanofiber for skin care applications. In contrast, DWCC uses a benign water-acid solvent system, which makes it easier to scale-up and manufacture (see Figure 1c) while also remaining safe for skin.
Besides the structural benefits of DWCC, it offers a unique advantage over gelatin in that it is dissolvable even at room temperatures or lower, even in cold water, which makes it easy to apply to the skin at any temperature (see Figure 1d).
Sonic Electrospinning
Electrospinning in general is a nanofiber fabrication technique that utilizes an electrostatic repulsive force within an electric field to stretch a polymer droplet under high voltage.19, 20 At this voltage, the highly conductive marine collagen solution (3.217 S/m) overcomes surface tension (220 mN/m) and the kinetic viscosity of the polymer (320 cst) to form a jet that is stretched to a collecting material. During this phase, the jet of collagen transforms to a chaotic whipping motion,20 which is influenced by the voltage, temperature and relative humidity to form a solidified collagen fiber on the collector substrate. Sonic electrospinning, used especially to construct MCN, is a proprietary technology with a design that encourages a stable jetting process for an extended period over a large surface area.
The morphologies of electrospun jets can be categorized into five regimes.21 At a particular regime, which starts with a jet fluctuation, the hydrodynamic interaction within the collagen solution, combined with the complex jetting mechanism in an electrically charged environment, leads the entangled DWC collagen alpha chain to stretch.
When the denatured polymer chain stretches, interactions between the molecular chains present in the solution also increase. Due to the high topological interactions in this regime, the HA present in this particular solution also stretches to form an inter-penetrated network with the collagen, resulting in increased molecular stability of HA. In the case of additional polyphenolic actives, e.g., the antioxidants from kiwi fruit and grape skins, which comprise gallic acid, proanthocyanins, catechins, and vitamin C, these are chemically bonded to the collagen backbone in the solution. Thus, during the spinning process, the collagen nanofiber carries the encapsulated/chemically bonded active compounds within the chains, protecting its potency and stability against photo-oxidation.
MCN Characterization
As noted, FTIR spectroscopy was used to analyze and assure the presence of polyphenol components in the sonically electrospun MCN (see Figure 2). Signature peaks specific to polyphenols confirmed they were bound to the MCN backbone. Thus, the encapsulation and protection of bioactives present in the MCN, afforded by the sonic electrospinning technique, becomes an essential component to enable the creation of a rapidly dissolving fabric for fast penetration.
Results also showed the diffusion behavior of actives does not prefer a single pathway for permeation.
Penetration Protocol
To measure the penetration of actives from MCN into pig skin, OCT was utilized. First, the test sample measurement sites and real-time measurement period, or rate, were established. The recommended timeframe of one hour (50 min) to scan and obtain all images and measurements was strictly followed.
The skin surface was wetted with room temperature water (~22°C) prior to placing the actives-embedded MCN onto it; all measurements were performed at room temperature.
The treated skin was then placed/mounted precisely under the probe, onto the OCT platform, where the bioactives-embedded MCN was placed, avoiding any additional compression of the sample. For each sample analysis, the OCT dynamic range was adjusted to avoid random noise and to guarantee optimal imaging with reasonable depth and good contrast. Software was used to analyze OCT data, and the images were reported as 2D or 3D image files, while the OCT signals’ strength in relation to the skin depth was reported in separate graphs and elaborated using additional software.
Permeability Results
When the MCN sample was placed on the skin surface, rapid diffusion behavior was instantly observed. Figure 3 shows the degree of penetration of the bioactives from each nanofibrous matrix. To optimize this visualization and provide a better understanding of the penetration behavior, each skin sample was arbitrarily subdivided into 256 smaller volumes during the OCT analysis, and each section was photographed and analyzed separately. As the OCT profiles reveal, the collagen nanofiber penetrated deeply, crossing the stratum corneum and reaching a depth of 1.5 mm, up to the dermal-epidermal junction (DEJ), almost immediately, i.e., at 0 min. This penetration continued further into the skin layers and reached a depth of 1.75 mm after 18 min.
It is documented that the size of a molecule and its lipophilicity are the major determinants of penetration processes through the stratum corneum. This means the permeability of a molecule is directly related to its hydrophilicity or lipophilicity, and inversely proportional to its molecular size. Many cosmetic or drug formulations use skin penetration enhancers to help promote the transport of actives into skin,22 with water being the safest and most effective skin penetration enhancer; it has a high permeability constant of 5.5 × 10-6 cm/hr.23 Therefore, simply hydrating the stratum corneum can modify its surface efficiently to promote actives penetration.
The DWCC nanofiber matrix, being a water-soluble material, naturally diffuses through the stratum corneum pores on a wet surface due to its hydrophilicity, which in turn promotes the transport of actives attached to it, as is evident from Figure 3a.
Continuing its penetration, over the next 35 min, MCN crossed 2 mm of skin, which is where the dermal-epidermal junction (DEJ) reaches the dermal layer. This zone between the dermis and epidermis is the cutaneous basement membrane—a dynamic interface where the two layers adhere to one another. The DEJ typically restricts the passage of most molecules, based on charge and size, between the epidermis and dermis1 but due to its structural advantages, MCN permeated the DEJ layer (see Figure 3b) and spread across the network.
Kiwi fruit and grape skin bioactives were delivered by the nanofiber to a depth of 2.5 mm or the demal layer of skin.
The actives also continued to penetrate further, reaching 2.5 mm—a dermal layer of the skin—after 50 min (see Figure 3c). This layer consists of fibroblast connective tissues that produce natural collagen and other fibers. Therefore, MCN, with the appropriate actives, could potentially be used to reconstruct the ECM of skin. Figure 4 shows the penetration level of the initial volume 1 and the final volume 256 at 0 min and 50 min. Note that all images showed a comprehensive bioactive profile across the whole sample and the clear absence of particle agglomeration. These results also show the diffusion behavior of actives does not prefer a single pathway for permeation.
Discussion
As previously described, the instant diffusion behavior was achieved by the breakdown of the soluble amino acid chain of the MCN, along with the HA molecule and bioactives, into small, nano-sized fragments in an aqueous phase that crosses the skin structures. Such an instant breakdown appears to be possible only through this high surface area-nanofiber matrix.
In comparison, triple helix collagen could not break down so rapidly, especially with water at room temperature, due to its high molecular weight. And HA, being a bulk polysaccharide, and hence classified as an injectable product, could not otherwise penetrate the skin. The observed success of the deeply penetrating actives is greatly associated with the electrospinning technique and the nature of soluble DWCC structure.
It is important to consider the diffusion characteristics of the ingredients are governed by both the skin type, i.e., its absorbing efficiency, as well as the working mechanism of the collagen nanofiber matrix. However, to some degree, the chemical nature of the actives also controls the diffusion behavior.
Conclusions
In both cosmetics and medicine, it is preferred that topically administrated actives act either dermally or transdermally. Unfortunately, most topically administrated drugs or actives cannot penetrate or permeate the skin layers without skin barrier manipulations. The key to driving the actives deeper into the skin is to design an intelligent delivery system that can carry those actives to where they can live up to their full potential and work within the skin for long-term effects.
The described marine collagen in a nanofiber matrix form, along with the inclusion of HA and natural bioactives, is shown here to provide one such intelligent skin delivery vehicle, which delivered actives in less than one minute. This exceeds all the literature values published to date for non-invasive delivery, especially as a natural product. The penetration also continued for an additional 50 min to reach 2.5 mm of a deep dermal layer of the skin, making it perhaps one of the fastest non-invasive active delivery systems in the world.
Further, the interfacial surface chemistry of the nanofiber matrix could be varied to encapsulate multiple actives, custom-designed for specific target applications, as well increase their dispersion and efficacy while decreasing formulation complexity. The technique could also potentially be used to send both water- or oil-soluble cosmetic ingredients or drugs into the skin without the use the emulsifiers, stabilizers and skin penetration enhancers.
Finally, the strong bonding of actives within the nanofiber matrix and rapid entry of those actives into skin illustrate its potential use in beauty facial masks and wound care. This process could potentially revolutionize the skin care industry and, importantly, has been shown to be 100% sustainable, reproducible, cost-effective and scalable to meet industry demand. In the future, clinical studies will assess the feasibility and efficacy of this system for dermatological applications.
References
- MMA Elsayed et al, Lipid vesicles for skin delivery of drugs: Reviewing three decades of research, Intl J Pharmaceutics 332(1) 1–16 (2007)
- US Pat 9775917B2, Nanofiber and bioactive compositions and related methods, IC Hosie, GF Vile et al, Active Fibres Ltd, (2017)
- N Boyera, I Galey and BA Bernard, Effect of vitamin C and its derivatives on collagen synthesis and cross-linking by normal human fibroblasts, Intl J Cos Sci 20(3) 151–158 (2001)
- Y Peng et al, Evaluation for collagen products for cosmetic application, Intl J Cos Sci 26(6) 313–313 (2004)
- A Alves et al, Cosmetic potential of marine fish skin collagen, Cosmetics 4(4) 39 (2017)
- N Zaman, Influence of the (ultra) structure of skin specimens used for pharmacological studies on the tissue permeability, Department of Pharmaceutics, Laboratory for Medicinal Chemistry, Universiteit Gent, Belgium (2014)
- IP Dick and RC Scott, Pig ear skin as an in vitro model for human skin permeability, J Pharma and Pharmacol 44(8) 640–645 (1992)
- E Abd, et al, Skin models for the testing of transdermal drugs, Clinical Pharmacology: Advances and Applications 8 163–176 (2016)
- J Welzel, Optical coherence tomography in dermatology: A review, Skin Res and Tech 7(1) 1–9 (2008)
- TH Silva et al, Marine origin collagens and its potential applications, Marine Drugs 12(12) 5881–5901 (2014)
- S Arvanitoyannis Ioannis and A Kassaveti, Fish industry waste: Treatments, environmental impacts, current and potential uses, Intl J Food Sci & Tech 43(4) 726–745 (2007)
- K Hofman et al, Effects of the molecular format of collagen on characteristics of electrospun fibers, J Materials Sci 47(3) 1148–1155 (2012)
- JE Eastoe, The amino acid composition of mammalian collagen and gelatin, Biochem J 61(4) 589–600 (1955)
- D Le Corre-Bordes, K Hofman and B Hall, Guide to electrospinning denatured whole chain collagen from hoki fish using benign solvents, Intl J Biological Macromolecules 112 1289–1299 (2018)
- K Hofman et al, High-throughput quantification of hydroxyproline for determination of collagen, Analytical Biochemistry 417(2) 289–291 (2011)
- HG Reinhard Schrieber, From collagen to gelatine, in Gelatine Handbook: Theory and Industrial Practice, Wiley-VCH Verlag GmbH & Co (2007) pp 45–117
- DI Zeugolis et al, Electro-spinning of pure collagen nanofibers—Just an expensive way to make gelatin? Biomaterials 29(15) 2293–2305 (2008)
- O Hartman et al, Biofunctionalization of electrospun PCL-based scaffolds with perlecan domain IV peptide to create a 3-D pharmacokinetic cancer model, Biomaterials 31(21) 5700–5718 (2010)
- B Kannan, H Cha and IC Hosie, Electrospinning—Commercial applications, challenges and opportunities, in Nano-size Polymers: Preparation, Properties, Applications, S Fakirov, ed, Springer International Publishing, Cham (2016) pp 309–342
- YM Shin et al, Electrospinning: A whipping fluid jet generates submicron polymer fibers, Applied Physics Letters 78(8) 1149–1151 (2001)
- Y Cai and M Gevelber, The effect of relative humidity and evaporation rate on electrospinning: Fiber diameter and measurement for control implications, J Mat Sci 48(22) 7812–7826 (2013)
- J Kalbitz, R Neubert and W Wohlrab, Modulation of drug penetration in the skin, Die Pharmazie 51(9) 619–637 (1996)
- RL Bronaugh, The effect of cosmetic vehicles on the penetration of N-nitrosodiethanolamine through excised human skin, J Inves Derm 76(2) (1981)