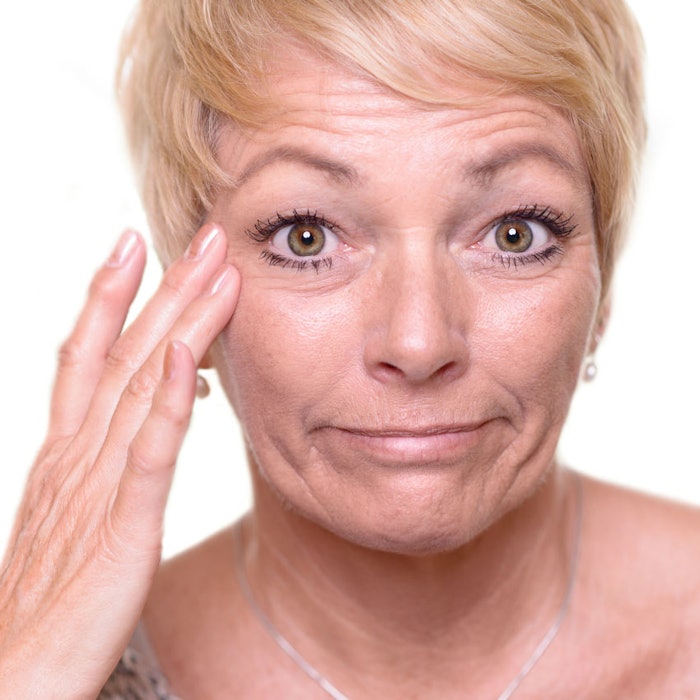
Editor’s note: Glycation has previously been addressed1 in terms of its impact on skin aging, and several ingredients as well as finished products making anti-glycation related claims have since appeared on the market.2–5 The present article, adapted from Draelos and Pugliese*, provides a review of the chemistry involved in the glycation process to assist formulators in developing topical or nutricosmetic solutions for mature skin care.
Glycation fortunately does not occur largely in the dermis before age 35 but once it begins, along with intrinsic aging, it progresses rapidly. These long-lived tissues are the target of advanced glycation end products (AGEs) that bind tenaciously to collagen and elastin, more avidly to elastin, which can be seen in the upper dermis in the realm of elastin. In fact, a paper by Mizutari et al. showed a section of a skin biopsy from the face of a 91-year-old revealing tangled masses of elastotic material in the upper dermis.6 Elastotic material consists of abnormal elastin fibers and protein and is usually associated with sun damage. It does not function like normal elastin and is quite stiff.
Many of the fine processes involved in glycation remain to be discovered. However, it is known that glucose and other simple sugars combine with proteins as a first step, and that sugars combine with amino acids and other compounds to initiate the process. As an example, fructoselysine, formed by glycation of the amino acid lysine, can be oxidatively cleaved to form smaller reactive compounds such as carboxy-methyl lysine (CML) and pentosidine.7
As consumers age they have a greater risk of glycation-initiated damage and their glucose level is the major culprit in initiating the formation of AGEs and glycation. Glucose, in its common closed-ring form, D-glucopyranose, is a rather unreactive molecule that is an important cellular fuel. It is the only sugar that circulates in abundance and although it is relatively harmless, as noted, it can become harmful through its transformation into an AGE by an intricate random process that occurs in animals and vegetables. This “browning” action requires no enzymes but depends merely upon temperature and an abundance of reactants.
As an AGE, glucose becomes molecular glue that makes blood vessels inelastic and stenotic. It provokes inflammation that in turn promotes hypertrophy of vascular smooth muscle and the extracellular matrix. These events are also responsible for atherogenesis, or hardening of the arteries, that differs in diabetics due to a quickened pace since there is a greater availability of glucose from the atherogenesis of normative aging. To better understand the process of glycation, it would first be helpful to review some sugar chemistry and the pathophysiology of glycation.
Sugar Chemistry
Sugars are carbohydrates that often are joined with proteins to form glycoproteins or other complex compounds. These essential biochemicals are made by specific enzymes for specific functions. Hyaluronic acid and chondroitin sulfate are examples of these glycoproteins. When sugar reacts with protein in a nonenzymatic manner, it forms AGEs that are harmful to the body. Whereas glycosylation is a normal, genetically controlled process that requires enzymes to produce the end products, glycation is a nonenzymatic process that is not programmed by genes and is not a physiological process that benefits the body.
Table sugar or sucrose is a disaccharide made up of two monosaccharides, glucose and fructose, bonded together via a glycoside linkage. This -C-O-C- group is formed when the two sugars come together and eliminate a molecule of water. Consuming table sugar is the same as eating a disaccharide, but before the body can use it, it must be broken down by enzymes to simple sugars that then are metabolized separately. Figure 1 shows the structure of a simple sugar, glucose. It has six carbon atoms in a straight chain but can spontaneously react to make a circular form, shown in Figure 2, which occurs when it is placed in water. Simple sugars may be aldehyde sugars and are called aldoses or ketoses, depending on the location of the carbonyl or the C=O group. In glucose molecules, the C=O group is on the first carbon, while fructose has the C=O group on the second carbon. Glucose is an aldose and fructose is a ketose.
Glucose and other simple sugars also may be linked to amino groups to produce amino sugars. For example, if an NH2 group was linked to glucose, the new compound would be called glucosamine. If linked to galactose, the new compound would be called galactosamine. An even more complex compound, found in nerve tissue, is acetyl galactosamine.
Larger than the disaccharides are the oligosaccharides, which form a group of less than 10 sugars linked together by glucosidic bonds, and polysaccharides such as starch and cellulose, which include more than 10 simple sugars linked together.
Rounding out the basic sugar nomenclature are the O-glucans and N-glucans. When a glucosidic linkage is found through an oxygen molecule, it is called an O-glucan, whereas a glucosidic linkage found through a nitrogen molecule is called an N-glucan. Figure 3 shows this relationship. Here, glucose is labeled as D-β-glucose and D-α-glucose, D referring to dextra or right, in Latin, and β and α referring to the configuration of certain hydroxyl groups or other groups on the sugar molecule. These designations are important as they have profound effects on how the molecule reacts with other substances.
Sugar Interactions: The Maillard Reaction
Building on the basic forms of sugar chemistry, this article now considers their interactions. There are at least five critical steps to forming AGEs, the first two of which are related to sugar content and are spontaneously reversible. The remaining reactions are permanent and have adverse pathological actions on the body, although they have been shown to be reversible, in part, by new chemical agents still in trial.
As is well-known, cooked meats change color when heated in a process known as browning, which imparts both flavor and aroma to cooked meat. Chemically, this process is the Maillard reaction, a nonenzymatic browning that involves the reaction of simple sugars, i.e., carbonyl groups, and amino acids—free amino groups. This reaction occurs at lower temperatures and higher dilutions than caramelization, which occurs in candy-making. Figure 4 shows the steps of the Maillard reaction, which follow several pathways depending on the type of reactants or ingredients, the temperature and the pH. The three main stages include condensation, degradation, and polymerization and browning, described here. The reaction in Figure 5 outlines the process using basic reaction groups. While the Maillard reaction is not completely understood, the basic reactions are well-known and important to understanding glycation.
Stage one, condensation: The Maillard reaction starts when the carbonyl group (HC=O) of the aldose and the free amino group of an amino acid (-NH2), which usually is a protein or peptide, combine to form an N-substituted aldosylamine; simply put, a sugar plus an amino acid. This basically is an amine-assisted dehydration reaction of sugar, meaning water is formed and the condensation product rapidly loses water as the product is converted into a Schiff base. This Schiff base contains a carbon-nitrogen double-bond with the nitrogen atom connected to an aryl or alkyl group (H-C=N-R).
The Schiff base then forms a ring structure. This reconfiguration, referred to as the Amadori rearrangement, forms a ketosamine as the molecular structure around the oxygen atom changes. If the aldose is glucose and amino acid glycine, then the Amadori product is 1-amino-1-deoxy-2-fructose, or monofructoseglycine (MFG). Amadori rearrangement is considered a key step in the formation of major intermediates for the browning reaction.
Stage two, degradation: Next, the Amadori product degrades by one of three main pathways, depending upon the conditions. Consider the Strecker degradation pathway (see Figure 6). In the Strecker degradation, the amino acids formed in the linkage undergo oxidative degradation by carbonyl compounds that arise from the degradation of ketosamines. In this degradation reaction, amino acids first react from Schiff bases and then undergo acid-catalyzed decarboxylation. The new Schiff base is easily hydrolyzed to form an amine and aldehyde.
Strecker degradation is characterized by the production of CO2 and the net result is a transamination reaction that may be important for the incorporation of nitrogen into melanoidins. Also, the aldehydes formed may be important as auxiliary flavor compounds and contribute to melanoidin formation. Strecker degradation products are implicated in “off” storage-related flavors.
Stage three, polymerization and browning: This stage is characterized by the formation of brown pigments and roasted and toasted aromas and tastes. The formation of melanoidins is the result of the polymerization of the highly reactive intermediates that are formed during the advanced Maillard reaction. These can be off or bitter flavors; off aromas such as burnt, onion, solvent, rancid, sweaty or cabbage; or positive aromas such as malty, bread crustlike, caramel, coffee or roasted. The chemistry of these compounds is not well-known but some of the reactions are included in the references.
Of all the amino acids, lysine results in the most color in the Maillard reaction due to its ε-amino group; cysteine results in the least color. Therefore, foods containing proteins that are rich in lysine residues such as milk proteins are likely to brown readily. The sugar-to-amino-compound ratio also influences the amount of color produced. For example, a glucose-glycine model system containing 65% water and stored at 65°C shows a rapid increase in color formation as the glucose-to-glycine ratio is decreased. In foods, attempts to prevent the reaction should be directed toward removing one of the reactants: the amino compounds for carbohydrate-rich foods or the reducing sugars for protein-rich foods.
Water is also produced during the Maillard reaction. Thus, as a consequence of the law of mass action, the reaction occurs less readily in foods containing water. In practice, the Maillard reaction occurs most rapidly at intermediate water content levels. In addition, low pH values, ≤ 6, favor the formation of Amadori rearrangement products but in practice, the effect of pH is not this clear cut. For sugar-glycine systems, the descending order of color development at pH levels lower than 6 was found to be: xylose > fructose > glucose > lactose > maltose; at a pH of 6, the order was: xylose > glucose > fructose > lactose > maltose. In Maillard browning, it appears that the rate of color development is not related directly to the structural stability of the sugars.
Harmful Effects of AGEs
As noted, the Maillard reaction starts by forming a Shiff base, then proceeds to form multiple chemicals called AGEs, which have adverse effects. For instance, AGEs can link up with proteins and denature or alter them to become nonfunctional, cross-linked collagens, which cause stiffness of the skin.
The actual AGE process begins when the D-glucopyranose ring spontaneously comes open in watery fluids. The aldehyde can react with an amino (NH2) group on a large biologic molecule, hooking the sugar onto a protein, for example. Physicians and diabetic patients are familiar with one specific glycation end product called hemoglobin A1c that is an Amadori product formed by glucose linking itself onto the β chain of normal hemoglobin.
Proteins are not the only targets, as lipids such as phosphatidylethanolamine and phosphatidylserine offer the amino groups required for initial linking of a sugar. AGEs also increase susceptibility of low-density lipoproteins (LDL) to oxidation. Further, glucose targets collagen molecules with its numerous amino groups, at any one of which AGE formation may begin, though an AGE can attach itself to more than one collagen. Collagen molecules usually slide freely, allowing vessels to dilate and contract but they become immobilized after attachment.
AGEs can also perturb cellular function by binding to a variety of receptors recently identified on various cell types, including macrophages, endothelial cells and smooth muscle, and renal cells, as well as neuronal cells. These receptors are called receptor-advanced glycation end products (RAGE). The RAGE receptor is a toll-like receptor, which is a key mediator in the body’s response to bacteria or viruses, for example. The AGEs, glycated proteins, lipids or even nucleic acids form a group of patterned ligands that can interact with RAGEs and initiate cellular signaling programs that include activation of NF-ĸB, a major inflammatory agent suspected in aging. Unlike binding directly to a long-lived protein such as collagen or elastin, these soluble AGEs act as ligands on RAGEs and stimulate the inflammatory process. RAGEs can sustain cellular activation and convert short-lasting pro-inflammatory responses into long-lasting cellular dysfunction.
Glycation Damage in Skin Cells
Two glycation end product carbonyls that are quite plentiful in the body are known as methylglyoxyl and glyoxyl. Recall that carbonyls are by-products of the early Maillard reaction and are reactive compounds. Figure 7 shows how both glyoxal and methylglyoxal can be produced from glucose without going through the whole Maillard reaction. These reactions proceed with oxygen, so antioxidants are not helpful in preventing them. These two end products have been found to interfere with biochemical substances and reactions, one in particular being DNA.8, 9 Both glyoxal and methylglyoxal damage DNA in intact cells; glyoxal is able to produce DNA strand breaks while methylglyoxal is responsible for DNA protein cross-linking that results in nuclear condensation and the inactivation of some sections of DNA.
Glycation has been found to cause adverse effects in keratinocyte function; for instance, it has been reported that glycation products diminish the effectiveness of collagen type I binding to keratinocytes, reducing the migration ability of keratinocytes in the epidermis. However, it does not affect the proliferative capacity of keratinocytes.10 This finding could relate to impaired wound-healing.
Glycation also has been connected to the accelerated aging of keratinocytes. For instance, placing keratinocytes in tissue culture with a solution of glucose at 100 mM, or glyoxal at 0.1 mM, will change the biological characteristic of the cells in three days from young keratinocytes to old, late-passage keratinocytes that show signs of slowing down, nearing death and unable to divide. This is significant because it indicates that a poor diet, high in sugars, can markedly speed up the aging process.11 These are but a few of the adverse effects of glycation on skin.
Prevention and Treatment
To prevent further glycation in the skin, controlling one’s sugar intake generally is suggested. In addition, some supplements are proposed to help to prevent glycation from occurring, including aminoguanidine, benfotiamine, pyridoxamine and carnosine. Aminoguanidine has a long use as a glycation inhibitor but as of late, there are some reports of low toxicity, mainly coupling with vitamin B-6.12 Benfotiamine is a lipid-soluble thiamine or B-1 vitamin, whereas pyridoxamine is a form of pyridoxine or vitamin B-6 that does not have the side effects of B-6 and has been shown to be an excellent glycation inhibitor.13
Carnosine is a dipeptide consisting of alanine and histidine, which has been shown to counteract free radicals and help prevent peroxidation of cell membranes. It can delay aging and rejuvenate senescent cultured human fibroblasts, which cannot be explained by its antioxidant, free radical- and metal ion-scavenging activities. It is well-known that carnosine reacts with small carbonyl compounds such as aldehydes and ketones, and thereby protects macromolecules against their cross-linking actions. As noted, aging changes are associated with accumulation of carbonyl groups on proteins and a recent study determined that carnosine reacts with protein carbonyl groups nonenzymically, a process termed camosinylation. Similar reactions could occur in cultured fibroblasts and in vivo. Initial experiments suggesting that carnosine is effective in vivo appear to relate to carnosine’s anti-glycating actions.
Although glycation has been known about for more than 20 years, the information is only now becoming available to the general public. Antiglycation agents are widely used in Europe to treat both diabetes and heart disease. In skin care, thus far, topical products on the market are few and not very effective; in relation, the extent of glycation in the skin can be assessed by measuring a fluorometric chemical called pentosidine in the skin. This material accumulates in a linear fashion over time, and a consumer can actually determine whether a topical product is effective at reducing its content in skin. This could open a new era of skin care and anti-aging treatments.
Conclusion
Glycation is the nonenzymatic joining of a sugar and a protein or a lipid. It is a process that occurs naturally in foods, especially when cooked. The Maillard reaction is one of these processes that starts by forming a Shiff base and proceeds to forming multiple chemicals called AGEs, which have adverse effects on biological processes. AGEs can link up with proteins and denature or alter them to be nonfunctional, cross-linked collagens, which causes stiffness of the skin. Skin collagen has a long half-life and such cross-linked forms do not go away; they are also not fully reversible at present. Elastin is another long-lived protein that is easily glycated and lasts a long time, and denatured elastin is associated with slackened skin. In the skin, glycation accounts for accelerated aging, yellowing and stiffness and decreased circulation. Skin cannot look young and healthy with glycation products and treatment is best started with prevention by diet control, reducing total calories and avoiding high sugar foods. Supplements such as aminoguanidine, pyridoxamine, carnosine and benfotiamine are excellent glycation preventors. As noted, topical products to treat glycation thus far are few and not very effective, although a recent approach measuring pentosidine in the skin suggests a new approach to skin care and anti-aging treatments.
References
- www.cosmeticsandtoiletries.com/research/ biology/7786471.html (Accessed Apr 13, 2011)
- www.cosmeticsandtoiletries.com/formulating/category/antiaging/96299259.html (Accessed Apr 13, 2011)
- www.cosmeticsandtoiletries.com/formulating/ingredient/active/8811677.html (Accessed Apr 13, 2011)
- www.cosmeticsandtoiletries.com/formulating/ingredient/active/92857824.html (Accessed Apr 13, 2011)
- www.cosmeticsandtoiletries.com/networking/news/company/114199839.html (Accessed Apr 13, 2011)
- K Mizutari et al, Photo-enhanced modification of human skin elastin in actinic elastosis by N(carboxymethyl) lysine, One of the glycation products of the Maillard reaction, J Invest Dermatol 108 797–802 (1997)
- DG Dyer et al, Accumulation of Maillard reaction products in skin collagen in diabetes and aging, J Clin Invest 91 2463–2469 (1993)
- MJ Roberts et al, DNA damage by carbonyl stress in human skin cells, Mutat Res 522 45–56 (2003)
- J Zeng et al, Evidence for inactivation of cysteine proteases by reactive carbonyls via glycation of active site thiols, J Biochem 389 196–205 (2006)
- K Moritra et al, Migration of kerartinocytes is impaired on glycated collagen type I, Wound Repair Regen 13 93–101 (2005)
- U Berge et al, Sugar-induced premature aging and altered differentiation in human epidermal keratinocytes, Biogerontology: Mechanisms and Intervention 1100 524–529 (2007)
- K-C Chang, Aminoguanidine prevents age-related deterioration in left ventricular-arterial coupling in Fisher 344 rats, British J of Pharmacol 142 1099–1104 (2004)
- JM Onorato et al, Pyridoxamine, an inhibitor of advanced glycation reactions, also inhibits advanced lipoxidation reactions, J Biol Chem 275 21177–211884 (2000)