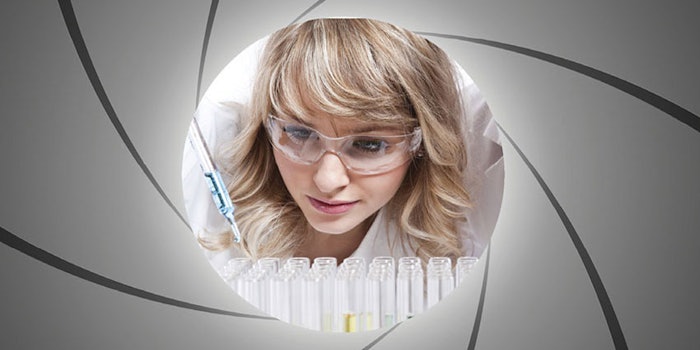
Editor’s note: Today’s consumers are highly focused on improving their general health and wellness. As such, cosmetics and personal care products have aligned with this driver and become more health-orientated. The rise in dermocosmetics is a perfect example. As a result, cosmetic companies are focusing innovation efforts in this direction. Here, we continue a longstanding discussion on fluorine chemistry. Borrowed from the pharma world, it holds interesting basic concepts for cosmetics and personal care.
The pharma industry is a good example of the importance of fluorine chemistry (25–30% of new drugs contain fluorine) and of the transfer of concepts from an industry to cosmetics. This article begins with basic theories to illustrate the mechanisms, unknown to many, of oil/water repellency and insolubility behind fluorine chemistry; it then goes on with advanced discoveries in medicinal chemistry to define concepts for the formulation of cosmetics.
Look Within: Intramolecular Forces
Usually, the molecular structure of a substance offers valuable indications regarding its properties and, roughly, its behavior in formulation and application. However, for the correct evaluation of chemicals containing fluorine atoms, some considerations at the molecular level are necessary.
For example, despite their differences in chemical structure, perfluorotripropylamine and perfluorodecalin can be components of emulsions used as synthetic blood since they are similar in chemical thermal and biological stability, insolubility, and capability to carry respiratory gases. These unexpected similarities can be explained by two types of attractive forces, intramolecular and intermolecular, which make perfluorotripropylamine and perfluorodecalin homologues in formulation and application.
In a molecule, atoms are held together by intramolecular forces that determine how it can be modified and to achieve better stability. For example, a fluorine atom can substitute and mimic a covalently bound hydrogen atom with little change of the steric bulk of the molecule. This fluorine-hydrogen shift produces stronger fluorine-carbon intramolecular bonds due to fluorine, the most electronegative among all the atomic elements.
Therefore, the substitution of one hydrogen atom with a fluorine atom or a trifluoromethyl group is a route to improve the stability of drug molecules. Similarly, by substitution of all hydrogen atoms with fluorine atoms, very stable perfluoromaterials are produced, including two families of liquid materials, such as perfluoropolyethers (by far the most important for cosmetics) and perfluorocarbons (that have been added in this article mainly for speculative reasons since too volatile for a general use in cosmetics).
However, when perfluoropolyethers (PFPEs) and perfluorocarbons (PFCs) contact other molecules, their interesting properties are not explained intramolecularly; other forces determine their behavior and are essential in formulation and application.
Between Us: Intermolecular Forces
Generalities: Macroscopic observations point out the existence and action of attractive forces between neighboring molecules of a liquid or solid, referred to as intermolecular forces. Though much weaker than intramolecular forces, intermolecular forces determine important physico-chemical properties of polymers and substances. They are the glue that brings and holds molecules together; when a solid melts or a liquid boils, the intermolecular forces are broken while the intramolecular forces remain intact. Without them, molecules of a substance would move apart from each other and the substance would become a gas.
Intermolecular forces depend on the global polarity of a molecule and on the repartition of this polarity in the molecular structure. In the case of non-polar molecules (by definition, those without permanent dipoles), there is only one type of intermolecular force, which is created by the attraction of temporarily induced dipoles produced by the continuous motion of electrons. Such forces are referred to as dispersion or London forces.
In polar molecules, where permanent dipoles are present, in addition to dispersion forces, there are two other main types of intermolecular forces: between a permanent dipoles and temporary-induced dipoles, named also Debye forces, and between permanent dipoles, named Keesom forces. These specific denominations are not always respected and all intermolecular force are also named collectively van der Waals forces.
The generic assertion that dispersion forces are weaker than forces related to permanent dipoles is misleading. On the contrary, dispersion forces are more influential in the majority of substances and polymers. Present in all molecules, polar and non-polar, these forces vary enormously from one molecule to another, depending mainly on the size (i.e., molecular weight) and shape (i.e., straight or branched structure).
PFPE and PFC specifics: PFPEs and PFCs present a paradox, because their intramolecular forces are strong while intermolecular forces are weak. However, at molecular level, very weak does not mean negligible; quite the contrary.
On the whole, these molecules are non-polar since the (Cδ+–Fδ-) dipoles of the carbon-fluorine bonds, organized by attraction of head to tail, cancel each other out, with the net sum of zero. Further, the polarity of single intramolecular bonds does not match the high difference of electronegativity between fluorine and carbon. This is due to the low polarizability of fluorine atoms (see Table 1).
The low polarizability corresponds with minimum levels of intermolecular forces, 100% of the dispersion type, even in the case of high MW. This explains the similarity between perfluorodecalin and perfluorotripropylamine, in contrast with corresponding hydrogenated compounds. In other words, a perfluoroamine is first a perfluorinated substance and then an amine.
More generally, this mechanism explains the main properties of PFPEs and PFCs, including opposite properties such as hydrophobicity and lipophobicity, or homophobicity (self-repellency and insolubility); the molecules of any other type prefer to interact with themselves rather than with molecules of almost negligible intermolecular forces.
It is expected that these intermolecular forces determine not only some intrinsic properties of PFPEs and PFCs, but also their behavior in formulation. However, this occurs only partially, since there is a certain dependence on the other components of the formulation. This dependence increases with their polarity, suggesting the action of other types of intermolecular forces in addition to dispersion forces. The importance of this aspect and of the disproportion between causes and effects is such that a discussion is necessary.
The generic assertion that dispersion forces are weaker than forces related to permanent dipoles is misleading.
Hydrogen Bonds and Fluorine: A Cosmetic Theory
Cosmetics typically are complex mixtures of substances and polymers having certain purposes as far as performance and presentation. Their complexity increases when PFPEs and PFCs are present, which is due to discrepancies in expected and realized results. These discrepancies are not always evident and easy to explain. Unexpected phenomena, e.g., the stability of formulations, aggregation of molecules, and dissolution (of chemically modified PFPEs) in aqueous or oily phases, occur that would suggest interactions between molecules of fluorinated and non-fluorinated substances.
However, in cosmetics, observations and investigations of what happens at molecular level are simply impracticable. Cosmetic formulators typically learn and build their skills by practice and application, rather than theoretical concepts and laws. Many hardly have the time or opportunity (or interest) to learn the basics of fluorine chemistry and systematically combine perfluoromaterials with differentiated cosmetic materials to see their behavior in simple model compositions.
This divergent type of research offers opportunity for discovery but theory is needed to interpret the results and compare them with the initial expectations. This theory may lie in a special category of intermolecular bonds—hydrogen bonds, in addition to halogen bonds; the latter are only described here in brief for simplicity’s sake as they are similar to hydrogen bonds.
In contrast with cosmetics, the intermolecular forces in medicinal chemistry are experimentally and thoroughly investigated, even when they are very weak. This is due to their role in the contact of a drug molecule with a biological target. As noted, more attention is currently being dedicated to molecules having one or more hydrogen atoms replaced by a fluorine atom, which in doing so, produces permanent polarity and new intermolecular attractions. These interactions should be classified as Keesom forces for the presence of permanent dipoles, but they are named hydrogen bonds (H-bonds) because hydrogen atoms are involved.
Because of its importance in numerous natural processes and its prospects in supramolecular chemistry, today, the H-bond is the most analyzed non-covalent interaction. It is a bridge (–R–H∙∙∙Y–Z) of a polarized hydrogen atom shared between an atom, R, to which it is covalently bound (R–H) that works as a donor, and another atom, Y, to which it is electrophilically attracted (H∙∙∙Y), which works as an acceptor. Usually Y is a nitrogen atom or an oxygen atom. After a decades-long controversy, it is also now widely accepted that covalently bound fluorine atoms may work as proton acceptors with oxygen (–O–H∙∙∙F–C), or nitrogen (–N–H∙∙∙F–C) atoms as proton donors in the formation of H-bonds (see Pioneering the H-Bond).
From Drugs to Cosmetics
Covalently bound fluorine atoms of drug molecules are usually few and not always identical, with differences due to the three-dimensional conformation of the molecule, the positioning of fluorine atoms in the molecule, and the molecular context. These differences have a great importance in medicinal chemistry, as illustrated by Bissantz: “The same interaction may [be worth] a different amount of free energy in different contexts, and it is very hard to find an objective frame of reference for an interaction, since any change of a molecular structure has multiple effects.”13 For these reasons, the orientation to the target (often a protein) cannot easily be predicted and demonstrated.
This means, in the context of drugs, fluorine chemistry is usually not given the right conditions to make what is referred to as positive cooperativity; meaning that although a single interaction is quite weak, several simultaneously acting interactions increase effects well beyond their sum.
The situation is quite different when a PFPE or a PFC is in the context of a cosmetic formulation, for several reasons. These include:
- A much higher fluorine content, from 68% (for PFPEs) to up to 76% (for PFCs);
- Multiple fluorine atoms attached to a skeleton (usually linear), with the same (or similar) positioning as such that all fluorine atoms can have the same (or similar) type of contact;
- A high content of hydrogen atoms, including hydrogen atoms of hydroxyl groups with accentuated protonic character, which are more suitable for H-bonds; and
- Simple identification of the partner atoms (hydrogen atoms), which can even be regulated through the formulation or procedure (a key difference that never occurs in biological systems).
Certain visible manifestations can be ascribed to the positive cooperativity of H-bonds with the involvement of fluorine atoms, consisting in the fact that despite the weakness of a single bond, multiple interactions with hydrogen atoms of oils or glycerol (or other polyols) explain the unexpected stability of three-phase o+f/w emulsions;14, 15 or of glycerol dispersions containing a PFPE.16, 17
The favorable conditions of cosmetics to the formation of H-bonds involving fluorine atoms suggested to investigate PFCs added to model formulations representative of the investigation carried out previously on PFPEs. Despite absolute insolubility and high density, very stable compositions, on the shelf and at centrifugation, were the result of this investigation.18
Table 2 shows a list of fluorinated materials that have a similar stability behavior in cosmetic formulations despite their different chemical structures.
Sufficient experimental confirmations exist to apply the theory to a long list of fluorine-containing materials having widely differentiated chemical structures. This list includes:
- Linear, branched and cyclic PFPEs within a wide range of molecular weights;
- Fluoro-ether-alcohols and fluoro-ether-carboxylic acids, and their derivatives; and
- PFCs of various structure: cyclic (e.g., perfluorodecalin), condensed polycyclic (e.g., perfluoroperhydrophenantrene) and pseudo-cyclic (e.g., perfluorotrispropylamine).
This list might also include chemically modified PFPEs, but much more interesting prospects in formulations and applications are offered for these materials in solution form.
Intermolecular forces only partly determine PFPE and PFC properties. Other components in formulas also influence their behavior.
Chemically Modified PFPEs in Solution
Considering the insolubility of alkaline fluorides in water and of small molecules of PFCs in oils, which are independent from the nature of fluorine bonds (ionic or covalent), molecular weight (MW) or dissolving media (polar or non-polar), it would seem impossible to create aqueous or oily solutions with a fluorine content of up to 10-15% by dissolving a polymer with a high fluorine content. Unexpectedly, however, both solutions can be prepared with high levels of a chemically modified α,ω-Z-PFPE. This is a surprising fact, from speculation, formulation and application viewpoints, and requires further discussion.
Hydrophilic modification: The substitution of a single fluorine atom of a trifluoromethyl group with a hydrogen atom (–CF3 → –CHF2) may hardly be defined as hydrophilic modification, which appears negligible in reference to high MW. However, it has remarkable consequences when it concerns the two ends of a Z-perfluoropolyether chain. There is an evident cause and effect disproportion; the small CH group, inert and only slightly polar (the Δ electronegativity between carbon and hydrogen is only 0.35), which is typically present in hydrocarbons (i.e., non-polar substances), is able to influence the behavior of a much bigger molecule. Thanks to this small polarity, dihydro-Z-PFPEs, with a MW in the 500-800 range, are soluble in ethanol. Replacing the hydrogen atom with a more polar hydroxy group, the solubility is extended to a higher MW (up to 1500).
Therefore, the expectation is that a phosphate group (i.e., groups more polar than a hydroxy group) should work even better as the dissolving group. And indeed, not only concentrated solutions of Z- PFPE diphosphate in ethanol can be prepared, but it is also possible to dilute these solutions with the gradual addition of water in large proportion. By careful neutralization of phosphate groups, it is even possible to obtain direct dissolution in water.19
The dissolution of Z-PFPE diphosphates in aqueous systems is due to the cumulative interactions (H-bonds) between the molecules of the Z-PFPE chain and the molecules of ethanol/water (or simply water), with the additional contribution of attractions due to the phosphate groups. These interactions outweigh the separate interactions between the molecules of the dissolving media, reaching the conditions to have dissolution.
Interestingly, the low dispersion forces between the molecules of the perfluorinated chain, described earlier, cause this behavior, which is demonstrated by two experimental facts: first, the increase in MW of the PFPE chain does not produce the expected decrease in solubility, as occurs with hydrogenated chains; second, comparable structures (e.g., alkyl phosphates) are much less soluble, even in the case of significantly lower MWs. How can this occur, considering PFPEs are completely insoluble and that H-bonds involving fluorine atoms are weaker than common H-bonds?
Recall that intermolecular forces in PFPEs are 100% weak dispersion forces, due to induced dipoles of poorly polarizable fluorine atoms. Therefore, PFPEs are insoluble in all non-fluorinated substances since the interactions between the molecules of PFPEs with molecules of other substances are never able to outweigh the interactions between these molecules, irrespectively of their characteristics. But because the intermolecular forces of PFPEs are weak, the effects of H-bonds and of the chemical modification are relatively enforced, both quantitatively and qualitatively.
Quantitatively, with an overall remarkable increase in intermolecular forces due to the addition of stronger intermolecular forces of the permanent dipoles. Qualitatively, with the corresponding reduction in contribution of dispersion forces. This reduction has unexpectedly disproportionate effects on solubility. In the context of Z-PFPEs, there are decisive contributions from their double functionality and the allocation in the α-ω positions of the PFPE chain.
Therefore, there is an apparent paradox concerning PFPEs since two opposite extreme behaviors, i.e., insolubility and high solubility, are ultimately caused by the same dynamics. All unmodified PFPEs are insoluble due to “very weak dispersion forces,” while chemically modified α,ω-Z-PFPEs are “highly soluble”—or, more accurately, can be dissolved using special procedures and with the potential to incorporate higher concentrations of solutions than the corresponding non-fluorinated substances—since the “very weak dispersion forces” can be outweighed by intermolecular forces consisting of H-bonds with fluorine atoms, combined with the attractions due to the permanent polarity produced by chemical modification.
From an energetic view, there are three different levels: 1) PFPEs as such, with 100% weak dispersion forces only; 2) PFPEs in aqueous formulations, with dispersion forces and H-bonds; and 3) hydrophilically modified α,ω-Z-PFPEs in aqueous solutions, with dispersion forces, H-bonds and attraction forces generated by the chemical functionality.
Substituting a fluorine atom with hydrogen is hardly a hydrophilic modification but the effects are remarkable.
Lipophilic modification: Similar reasoning can be adopted to explain the dissolution of lipophilically modified α,ω-Z-PFPEs in oils. Only minor adjustments of the theory are necessary regarding the ratio between perfluorinated and lipophilic chains and the polarity of the oils.20, 21 The fact that polarity, e.g., esters vs mineral oils, is decisive for the dissolution of Z-PFPE dialkylamides suggests a key role of fluorine bonds involving oxygen atoms (carbonyl groups) of esters or similar oils. In the context of this article, it is enough to mention this type of fluorine bond, which is usually associated with the behavior of the other halogens; hence the collective name of halogen bonds.22
Conclusions
PFPEs were initially proposed as insoluble liquids to produce three-phase emulsions characterized by the coexistence of two discrete internal phases dispersed in one continuous phase.23 Some authors further developed the concept of multiple internal phases with the combined use of PFPE and silicone oils, to create more complex emulsions containing up to five phases.24
The use of PFPEs in cosmetics has allowed important improvements in emulsions, particularly moisturizing, stability and sensorial properties. Nevertheless, little attention has been dedicated to a theory explaining the increase in stability due to ingredients that should work against it, on the basis of Stokes’ law, in consideration of the high density of PFPEs, around 1.9 g /mL.
This article gives theoretical support to what is already a reality of industrial importance. Besides, it intends to introduce a novel area of development, paradoxically with great potential in monophase systems, in addition to various types of emulsions, including multiple emulsions, based on new mechanisms. In relation, it is necessary here to note that this development cannot be reduced simply to a concept of “soluble PFPEs”, apparently destructive of the most typical properties of PFPEs, i.e., water/oil repellency.
Within these materials, the shield of fluorine atoms opposes resistance to attack by any dissolving media, and there is strong conflict between the major part of the molecule that repels water and oils, and the minor part that loves water or oils. This means that the properties of oil/water repellency of PFPEs are not only retained, but their delivery to the finished product is unexpectedly much more important, while it is asymmetric depending on the chemical modification.
At any rate, it is important to make the distinction between dissolution (a kinetic process quantified by mol/min), and solubility, which represents the state at the equilibrium (a thermodynamic condition quantified by mol/g). It is critical to take this distinction into account either in formulation, with special attention dedicated to the procedure, and application. Therefore, in the case of chemically modified α,ω-Z-PFPEs, it is always more correct to use the term dissolution, even it is actually possible to prepare perfectly transparent concentrated solutions.
Describing the procedures for the formulation and potential application of chemically modified α,ω-Z-PFPEs is well beyond the objective of this article. However, it is possible to provide two key references, including: a vision for the chemical functionalization of PFPEs,25 and a recent important development for this concept in application.26
Presently, only one specific perfluorinated chain is used as a building block in the design of chemically modified PFPEs that are soluble in water or in oils. This is likely due to constraints in chemical synthesis and poor attention dedicated to this chemistry. However, it provides a strong opportunity to leverage the potential impact of fluorine chemistry in cosmetics, simply exploiting the typical cosmetic chemistry already applied to fatty and polysiloxane chains, along with the choice between aqueous and oily phases. Concisely, it is possible to show graphically the strong divergence of this area of development (see Figure 1).
References
- L Hunter, The C-F bonds as a conformational tool in organic and biological chemistry, Beilstein J Org Chem 6 38 4-5 (2010)
- W Johnson, Invitation to Organic Chemistry, Jones & Bartlett Learning, Burlington, MA, USA (1998)
- L Pauling, The Nature of the Chemical Bond, Cornell University Press, Ithaca, NY, (1939 and 1960)
- E Arunan et al, Defining hydrogen bond: An account (IUPAC Technical Report), Pure Appl Chem 83/8 1619-36 (2011)
- J Dunitz and R Taylor, Organic fluorine hardly ever accepts hydrogen bonds, Chem Eur J 3(1) 89-98 (1997)
- HJ Böhm et al., Fluorine in medicinal chemistry,ChemBioChem 5 637-643 (2004)
- P Jeschke, The unique role of fluorine in the design of active ingredients for modern crop protection, Chem Bio Chem 5 570-581 (2004)
- C Casorati, S Sciabola and G Crucia, Hydrogen bonding interactions of covalently bonded fluorine atoms from crystallographic data to a new angular function in the GRID force field, J Med Chem 47 5114-25 (2004)
- HF Bettinger, How good is fluorine as a hydrogen-bond acceptor in fluorinated single-walled carbon nanotubes, Chem Phys Chem 6 1169-1174 (2005)
- D O’Hagan, Understanding organo-fluorine chemistry: An introduction to the C-F bonds, Chem Soc Rev 308-19 (2008)
- Y Luy et al, Ab initio investigation of halogen bonding interactions involving fluorine as an electron acceptor, Chem Phys Lett 449 6-10 (2007)
- P Zhou, J Zou, F Tian and Z Shang, Fluorine bonding—How does it work in protein-ligand interactions, J Chem Inf Model 49(10) 2344-2355 (2009)
- C Bissatz, B Kuhn and M Stahl, A medicinal chemist’s guide to molecular interactions, J Med Chem 53/14 5061-84 (2010)
- S Bader et al, Three-phase emulsions: Perfluoropolyether-oil-water, Cosm & Toil 101(11) 45-8 (1986)
- F Brunetta, L Montesi, M Guarneri and G Pantini, Low molecular weight PFPEs: A new range of perfluorinated oils for cosmetics, Proceedings of the 18th IFSCC Congress, Venice (1994)
- G Pantini, F Brunetta and V Guidolin, Perfluoropolyethers: Status and new developments, Cosm & Toil 106(10) 71-80 (1991)
- F Brunetta and G Pantini, Multiple emulsions comprising a perfluoropolyether, SÖFW J 11 (1993)
- Unpublished investigation
- G Pantini, R Ingoglia and F Brunetta, PFPE phosphate: A multifunctional material, Cosm & Toil 114(8) 81-92 (2001)
- G Pantini, R Ingoglia, F Brunetta, A De Ascentiis and F Bernardi, Perfluoropolyether amide, Cosmet Technol 4(3) 27-32 (2001)
- G Pantini and M San Miguel, Perfluoropolyether amide, 15th IFSCC Congress, Buenos Aires (2001)
- G Resnati et al, Halogen bonding after 200 years, 1st International Symposium on Halogen Bonding, Porto Cesareo, Italy (June 18-22, 2014)
- F Brunetta, S Bader, G Pantini and M Visca, Three-phase emulsions: Perfluoropolyether-oil-water, Cosm & Toil 101(11) 45-49 (1986)
- A Bevacqua, K Lahanas, I Cohen and G Cioca, Liquid crystals in multiple emulsions, Cosm & Toil 106 53-6 (May 1991)
- G Pantini and R Ingoglia, Hydrophilically and lipophilically modified perfluoropolyethers as ingredients of non-conventional cosmetics, Proceedings of the IFSCC Conference, Seoul (2003)
- www.cosmeticsandtoiletries.com/formulating/category/skincare/Problems-with-Skin-Protectants-Part-III-New-System-Sets-the-Rules-371399401.html (Accessed Apr 1, 2017)