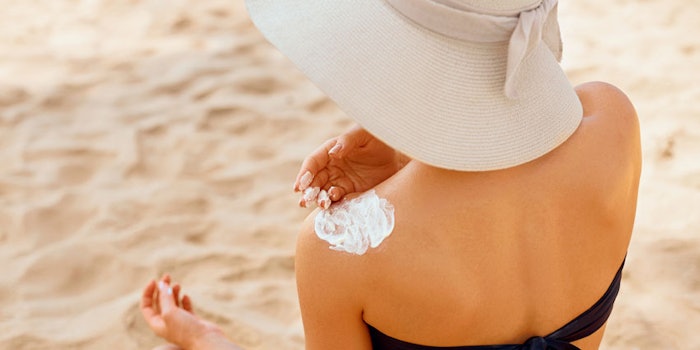
Due to a rise in melanoma and other skin related diseases,1–3 protecting the skin from the deleterious effects of the sun has become a priority in recent years. The quest for complete protection from the sun has led numerous research efforts for not only effective sunscreens, but also products that are extremely efficient in providing higher SPFs with lower levels of UV filters.4 The effectiveness of sunscreen products is typically denoted in terms of sun protection factor (SPF) and this value can be altered in a variety of ways including the specific sunscreen agents chosen or the type of delivery system or formula employed.
Sunscreen products work based on the ability of sunscreen actives to absorb photons in the UVB and UVA range. Simply put, according to Beer’s Law5, 6 the absorbance of light passing through a liquid is directly related to the concentration of the absorbing material in the liquid. However, considering the absorbance levels for US-approved sunscreen actives (see Figure 1), one can readily deduce that the actives must be utilized at many times more than would be expected in order to obtain the desired SPF.6 This difference can be attributed to several factors such as application uniformity,7, 8 complete UVB and UVA spectra coverage,9 formulation photostability10 and formulation polarity;11 in addition, one crucial factor is the solvent system used in the sunscreen formulation.12, 13
The Impact of Solvents
Again, a useful and efficient approach for improving the SPF and absorption of sunscreen actives is to modify their environment, i.e., the solvent in which they are placed. This modification can have a drastic effect on the overall performance of the sunscreen, ranging from SPF, excitation wavelength, polarity and product uniformity. Typically, organic sunscreens absorb UV radiation by promoting electrons into an excited state and their efficacy in doing so is based on a couple of factors: the amount of energy (wavelength) required to promote an electron, and the duration for which the electron remains promoted before returning to its ground state.
There are several ways that electrons can return to the ground state. One is by releasing the stored energy all at once and emitting a different frequency of energy (fluorescence). Others are by transferring their excited electron(s) to another molecule or by dissipating the energy as thermal heat. Each of these paths is directly related to the solvent in which the active ingredients are contained.12, 13 For example, as is well-known, butyl methoxydibenzoyl- methane or avobenzone, a common organic sunscreen, undergoes keto/enol tautomerization. When avobenzone is placed in a polar environment such as alcohol, the equilibrium lies heavily on the enol side. This results in a boost of the UV absorbance of avobenzone.
Solvent molecules can also transfer hydrogen atoms with excited molecules and trap them in a nonexcitable state. This leaves the active sunscreen unable to promote an electron and therefore unable to absorb UV radiation. For instance, Beyer et al.14 used Raman spectroscopy to show that the excited state of N,N-dimethyl-p-amino-benzoic acid can accept a proton from a polar solvent, which results in the loss of conjugation throughout the molecule. However, the interaction between the active ingredients and solvent can be modified to fit the product’s needs.
Employing Delivery Systems
Another effective way of optimizing the performance of organic sunscreens is to load them into delivery systems. Delivery systems most commonly involve amphiphilic systems, emulsions or amphiphilic macromolecules. Emulsions and amphiphilic macromolecules act in the same manner. In o/w emulsions, pockets of hydrophobic oil are contained in the core of micelles and when hydrophobic organic sunscreens are added into the emulsion, they migrate into the hydrophobic micelle cores and remain suspended in a unified matrix.
This organization provides two major advantages. First, the pockets of actives provide a boost in SPF. Second, the organization prevents the aggregation of the organics, in turn reducing the amount of color that appears on the skin during sunscreen application. Polymers as well as a new class of amphiphilic macromolecules, sorbeth 2 hexaoleate estersa, respond in the same way as emulsions, with the major difference being that the hydrophobic and hydrophilic portions of the formula are covalently attached in the same molecule or polymer chain.
As noted, sorbeth 2 hexaoleate esters are a newer technology and the subject of several recent patents.15–20 These compounds contain hydrophilic arms covalently bonded to a hydrophobic end group (see Figure 2), and this difference in polarity makes them soluble and effective when added into polar oil-based sun care formulations, as will be shown.
Materials and Methods
To evaluate the effects of solvents on the SPF values of sunscreens, a series of formulas (see Table 1) was prepared and their SPFs tested on the same subjects. Control products utilizing common solvents such as mineral oil, triglycerides, C12–15 alcohols benzoate and cetyl palmitate were made for SPF comparison purposes. SPF values were determined using a solar simulatorb with a continuous light spectrum in the UVA and UVB range (290–400 nm). The spectral output of the solar simulator was filtered to meet the spectral output requirements as outlined by the US Food and Drug Administration (FDA) and the International SPF Test Method.21–22
In general, formulas 1–5 were prepared as follows. First, A was dispersed and heated to approximately 70°C. B was then dispersed and heated to approximately 70°C. When both A and B were at 70°C, B was added to A with agitation. The combined AB was then cooled with agitation to approximately 50°C, and C was added to AB. For the oil formulas 6–13, all ingredients were heated as necessary until solution. Readers should note that Formulas 1–7 were prepared in the United States by a research consultant and tested in an independent lab. Formulas 8–13 were prepared internally and also tested by an independent European Lab.
Results
The SPF test results for formulas 1–13 are shown in Table 2. The use of sorbeth 2 hexaoleate in formulations had a dramatic effect on the SPF. The more polar esters, as compared with traditional solvents such as mineral oil, C12–15 alcohols benzoate, etc., provided SPF boosts as might be expected based on the previously described studies.12, 13 Specifically, in formulas having the same concentrations of actives, lower levels of the actives could be used to obtain the same SPF as formulations containing traditional esters when the test ester was present. In addition, an unexpected observation was the uniformity of the SPF values measured for the formulations made and tested by two different labs, one in North America and one in Europe. It is well-known that different test laboratories can obtain significantly different results on the same formula. With this in mind, it is important to note that the SPF results from Formulas 1 and 2; 3, 4 and 5; and 6 and 7, respectively, were obtained using the same test subjects. For example, the same five subjects tested by the same clinicians averaged an SPF of 19 with Formula 1 and an SPF of 32 with Formula 2. Likewise, Formulas 3, 4 and 5 were tested on the same five subjects, and Formulas 6 and 7 were tested on a different panel consisting of the same three subjects.
Results from Formulas 6 and 7 showed that on the same test subjects, Formula 7, consisting entirely of the test ester as the diluent, produced a much higher SPF than the similar Formula 6 made with the common oil ingredients octyl palmitate, mineral oil and C12-15 alcohols benzoate. Formulas 3, 4 and 5 showed similar results to Formula 2, suggesting that a broad range of sorbeth 2 hexaoleate percentages could be used to enhance SPFs.
Formulas 6 and 8 are practically identical in their components as were their SPF values—SPF 14.8 and 16, respectively, as determined by the two independent labs. Interestingly, Formulas 7 and 10 had practically identical SPFs, 29 and 30.2, respectively, but Formula 7 contained 91% sorbeth 2 hexaoleate whereas formula 10 contained 22.75% of the material. This suggests there is an optimal polar solvent level required to increase SPF, and that adding more polar solvent neither helps nor hinders the SPF.
Figure 3 graphically displays the SPF results for Formulas 8, 9 and 10, and these results suggest a linear relationship between increasing amounts of sorbeth 2 hexaoleate and SPF. If the slope were calculated, the SPF could be projected to continue increasing as the test ester is maxed. However, as noted, Formula 7 suggests there is a practical limit to the SPF increase and that with such a low level of sunscreens—i.e., 9%, the SPF would max out at about 30. Still an oil vehicle with 9% sunscreens having an SPF 30 is noteworthy.
Figure 4, showing the SPFs for Formulas 11, 12 and 13, suggests that with more sunscreen actives, the SPF increases with increased sorbeth 2 hexaoleate. It is interesting to note that if the optimum level of test ester has not been reached, it is more practical to gain higher SPFs by increasing the amount of sorbeth 2 hexaoleate than by increasing the percentage of sunscreen. For example, Formula 10, with 9% sunscreen actives and 22.8% test ester had an SPF of 30.2 whereas Formula 12, with the same ratio of actives at 15% and less test ester only produced an SPF of 26.8. This implies that for formulas with any level of active, it would be more cost-effective to optimize the level of sorbeth 2 hexaoleate based on the level of sunscreens.
Conclusions
The data shown here clearly demonstrates the impact of solvents on sunscreen SPF levels. It is known that different solvents with different polarities can not only shift the sunscreen absorbance, but also change the maximum absorbance, which can have a critical effect on SPF as well as the range of UV coverage. For example avobenzone was reported by Agrapidis13 to have a lambda max at 351 nm and an extinction coefficient of 27,200 in mineral oil, and a lambda max at 360 nm and an extinction coefficient of 31,100 in ethanol. Thus, in the polar solvent, the absorption is shifted to longer wavelengths and is increased by more than 10%. Therefore, theoretically, an ethanol formulation would provide both a greater SPF and broader coverage.
However, a problem occurs when the ethanol solutions are applied to the skin in that the ethanol immediately evaporates and the matrix is no longer polar. This problem is solved with the use of sorbeth 2 hexaoleate since it provides the polarity required but does not evaporate upon application. In formulas containing sorbeth 2 hexaoleate esters, a dose-dependent response was observed, up to an approximate SPF of 30 when the concentration of the test ester was altered and the amount of sunscreen was kept constant. This increase was significant and in some cases more than doubled the SPF of formulations not containing the test ester once it was added. It would be assumed this relationship would hold at both lower and higher SPFs.
Technologies such as the ester described here enable formulators to potentially change the shape of the industry since higher SPFs with less sunscreen actives will lower costs, irritation potential and concerns over sunscreen actives polluting the environment.27
References
Send email to [email protected].
1. L Lewis, Shedding light on sunscreens, MedEsthetics 21–26 (Mar/Apr 2011)
2. F Beddingfiel, III, The melanoma epidemic, The Oncologist 8 459–465 (2003)
3. A Jemal, S Devesa, T Fears and P Hartge, Cancer surveillance series: Changing patterns of cutaneous malignant melanoma mortality rates among whites in the United States, J National Cancer Institute 92 10 (May 17, 2000)
4. US Patent 7,309,481, Natural sunlight photostable composition, D Lott, assigned to Tanning Research Laboratories Inc (Dec 18, 2007)
5. Beer’s Law, available at https://en.wikipedia.org/wiki/Beer%E2%80%93Lambert_law (Accessed Apr 12, 2011)
6. J Lademann, A Rudolph, U Jacobi, HJ Weigmann, H Schafer and W Sterry, Influence of nonhomogeneous distribution of topically applied UV filters on sun protection factors, J of Biomedical Optics 9 6 1358–1362 (Nov/Dec 2004)
7. DL Lott, J Stanfield, RM Sayre and JC Dowdy, Uniformity of sunscreen product application: A problem in testing, a problem for consumers, Photodermatol Photoimmunol Photomed 19 17–20 (2003)
8. J O’Neil, Effect of film irregularities on sunscreen efficacy, J of Pharma Sci 73 7 888–891 (1984)
9. P Beattie, R Dawe, J Ferguson and S Ibbotson, Dose-response and time-course characteristics of UV-A1 erythema, Arch Derm 141 1549–1555 (Dec 2005)
10. C Bonda and D Steinberg, A new photostabilizer for full spectrum sunscreens, Cosm & Toil 115 37–45 (Jun 2000)
11. JW Wiechers, C Kelly, T Blease and J Dederen, Formulating for Efficacy, Cosm & Toil 110 50–61 (2004)
12. N Shaath, On the theory of ultraviolet absorption of sunscreens, J Soc Cosmet Chem 82 193–207(1987)
13. L Agrapidis-Paloympis and R Nash, The effect of solvents on the ultraviolet absorbance of sunscreens, J Society of Cos Chem 38 209–221 (July/Aug 1987)
14. L Beyer, S Yarasi and GR Loppnow, Solvent effects of sunscreen active ingredients using Raman spectroscopy, J Raman Spectrosc 34, 743–750 (2003)
15. US Pat 7,858,075, Spider esters in personal care applications, O’Lenick et al, assigned to SurfaTech Corp (Dec 28, 2010)
16. US Pat 7,723,456, Crosslinked silicone polymers based upon Spider esters, O’Lenick et al, assigned to SurfaTech Corp (May 25, 2010)
17. US Pat 7,569,607, Spider esters in personal care applications, O’Lenick et al, assigned to SurfaTech Corp (Aug 4, 2009)
18. US Pat 7,473,707, Spider esters in personal care applications, O’Lenick et al, assigned to SurfaTech Corp (Jan 6, 2009)
19. US Pat 7,462,729, Silicone spider esters in personal care applications, O’Lenick et al, assigned to SurfaTech Corp (Dec 9, 2008)
20. US Pat Application 20090253812, Spider Esters as delivery systems, O’Lenick et al, SurfaTech Corp (Aug 25, 2008)
21. Spectral output requirements for testing sunscreen drug products for over-the-counter human use, Proposed amendment of Final Monograph, CFR Part 352.70 (b) Light sources, Federal Register 72 165 (Aug 27, 2007)
22. International sun protection factor (SPF) test method, available at www.colipa.eu/ downloads/86.html (Accessed Apr 12, 2011)