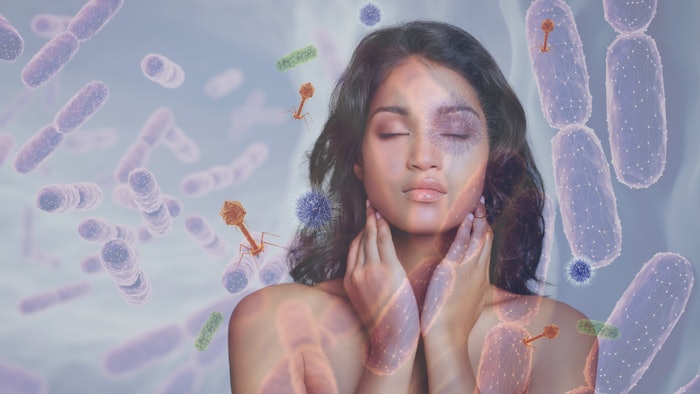
This article demonstrates how integrating WSG microbiome analysis with diagnostic criteria can help to uncover the underlying causes of skin-related issues. This leads to more precise classifications and personalized treatment. The article also presents a case study assessing the efficacy of a phage therapy to modulate the skin microbiome and, in turn, improve skin.
Log in to view the full article
This article demonstrates how integrating WSG microbiome analysis with diagnostic criteria can help to uncover the underlying causes of skin-related issues. This leads to more precise classifications and personalized treatment. The article also presents a case study assessing the efficacy of a phage therapy to modulate the skin microbiome and, in turn, improve skin.
The human skin hosts a diverse and complex community of microorganisms known as the skin microbiome, which maintains skin health by protecting against pathogens, modulating immune responses and preserving the skin barrier function.1 Advances in genomic technologies, particularly in sequencing methodologies, have significantly expanded our understanding of the skin microbiome, revealing intricate and site-specific variations across different body regions.2
Two primary approaches have been employed in microbiome research: 16S rRNA gene sequencing and metagenomic whole-genome sequencing (WGS). 16S rRNA sequencing, which targets a specific region of the ribosomal RNA gene, has been instrumental in identifying bacterial communities. However, it is limited in terms of a reduced resolution at the species level, inability to detect non-bacterial organisms, and lack of functional gene insights.3 These constraints can obscure the complexity of microbial communities, particularly in diverse ecosystems like the skin.
In contrast, metagenomic WGS allows for the identification and quantification of all microbial species, including bacteria, viruses, fungi and archaea. It also enables the analysis of functional genes, offering a detailed snapshot of the microbial landscape and its potential interactions with the host. This level of detail is vital to understanding the complex dynamics within the skin microbiome and the implications for skin health, disease and potential treatment.
The skin microbiome varies significantly between different regions of the body.
- The face, in particular, exemplifies this variation, with distinct areas such as the T-zone, cheeks and chin, each presenting unique environmental conditions that shape their respective microbial communities.3, 4
- The T-zone, characterized by higher sebum production, contrasts sharply with the relatively dry cheeks and the mixed characteristics of the chin.
- These site-specific differences are crucial for comprehending the overall function and health of the skin microbiome and for devising targeted therapeutic approaches.
A diverse microbiome is often associated with a resilient skin barrier and effective immune responses, while a less diverse microbiome may correlate with conditions such as acne, eczema and rosacea.3-5 In rare instances, diverse polymicrobial infections can be a hallmark of disease.3-6 The development of antibiotic-resistant strains within the skin microbiome is another critical aspect, complicating the treatment of skin infections and underscoring the need for alternative therapeutic strategies, such as phage therapy.7
Recent advances suggest that site-specific microbiome testing, combined with whole-genome sequencing, can aid in understanding these variations and open new avenues for treatment; for example, customizing a phage serum to target and control the overgrowth of identified pathogenic bacteria. Offering a precision-based solution to balance the microbiome could revolutionize skin care, managing skin issues more effectively while preserving beneficial bacteria essential for healthy skin.8
Materials and Methods: Measuring Microbe Diversity
Study design and sample collection: To investigate microbial diversity and composition across different facial sites, skin swabs were collected from 111 participants in three different areas: the cheek, chin and T-zone. A fourth swab of the ambient air environment was taken as a negative control and processed identically to other samples. Samples were collected using sterile swabs, which were immediately placed in sterile, DNA-free tubes with a non-toxic preservative buffer.
DNA extraction and sequencing: Microbial DNA was extracted from the swab samples using a standardized proprietary protocol optimized for skin microbiome analysis under aseptic conditions. Briefly, samples were preserved at time of collection, thoroughly lysed upon receipt in the laboratory, after which DNA was extracted and purified.
Sequencing libraries were prepared from total purified, fragmented DNA with minimal amplification. Libraries were then sequenced according to standard protocola, yielding short paired-end DNA sequencing reads. A short-read, high-throughput, whole-metagenome shotgun approach was used. These reads were pre-processed and clustered at a Jaccard distance of 0.06 to reveal 8 distinct clusters.
Data processing and clustering: Fastq files were pre-processed to remove human DNA and adapter sequencesa.9 Low-quality base pairs were trimmed and reads containing rare kmers were removed. Residual data was analyzed with proprietary software. Clusters were plotted on an MDS dimension-reduction scatter plot.9, 10
Microbial DNA quantification and hypothesis testing: Quantities of microbial genera on different skin sites were compared using the Wilcoxon Rank Sum Test. A two-sided test with unpaired samples was used, and continuity correction was applied. The null hypothesis was that the distributions of both groups were the same. p Values less than 0.05 were considered statistically significant (*); less than 0.01 as very significant (**); and less than 0.001 as highly significant (***).
Case Study: Divergent Clustering
In sub-analysis, one participant from the original test group exhibited a divergent clustering pattern and was thus selected for a detailed case study (Participant A). The microbial DNA concentrations and taxonomic composition of this individual's cheek, chin and T-zone samples were analyzed separately to investigate the underlying causes of the atypical clustering.
The presence of folliculitis in the T-zone was confirmed through clinical examination, and its impact on the local microbiome was assessed through comparative analysis with other participants. Results are described in the next section.
Case Study: Seborrheic Dermatitis and Phage Treatment
Another participant (Participant B) from the original cohort was a male in his 50s afflicted by highly visible, persistent seborrheic dermatitis with a decades-long history on the forehead. He submitted to a specified skin swab testb at the authors’ company labs in June 2023. The test was designed to assess skin’s condition and provide product recommendations. Based on the results, the individual was prescribed a Staphylococcus-specific phage serumc, as S. aureus is a bacterium usually linked to atopic dermatitis.
Participant B routinely applied the serum topically for approximately one year, following which he submitted to a second skin swab testb in June 2024. Measurements were taken from the forehead prior to phage treatment in June 2023 and in June 2024, after nearly one year of regular topical phage application.
Microbiome DNA sequencing analysis was conducted as described above, except the identity of the Staphylococcus-specific phage in the June 2024 test was confirmed by mappinga short reads to the genome sequence of the phage in the test serum with the bwa mem algorithm.18
Results: Microbial Clustering Across Facial Skin Sites
The analysis of microbial communities across different facial skin sites (cheek, chin and T-zone) from the 111 participants revealed eight distinct clusters (see Figure 1, below). The majority of samples (clusters 1-5) formed large groups, each containing between 29 to 96 metagenomes, indicating common microbial compositions shared among many individuals. Cluster 1 was the largest with 96 metagenomes while Cluster 8 only contained one metagenome. Clusters 6-8 were composed of a small number of samples (1-7 samples each), representing highly distinct microbial communities.
Notably, samples from the same individual are typically clustered together, suggesting that microbial genetic diversity is shared across different regions of the face. This was the case in the present study. For most individuals (54%), metagenomes from all three skin sites belong to the same cluster, which agrees with other studies indicating diversity between individuals is greater than microbial diversity found on a single individual. These findings also align with previous studies that emphasize the inter-individual diversity of the facial skin microbiome.12
Results: Differential Microbial Concentrations by Skin Site
The median DNA concentrations of common microbial genera varied significantly between the facial skin sites (see Figure 2, below). Lipophilic microbes, such as Corynebacterium, Cutibacterium and Malassezia, were notably enriched in the sebum-rich T-zone, consistent with the high lipid content of this area. Staphylococcus exhibited significant variation across the three skin sites, with the highest concentrations observed in the T-zone and the lowest in the cheek, correlating with the sebum levels.
Additionally, Streptococcus showed slightly higher DNA concentrations on the chin compared to the cheek, possibly due to the proximity of the chin to the oral microbiome, where Streptococcus is abundant. These findings corroborate the site-specific nature of the facial skin microbiome, with each skin site hosting a distinct microbial community.
Results: Divergent Clustering and Folliculitis Case Study
As stated, Participant A’s T-zone microbiome clustered separately from their cheek and chin samples (see Figure 3, below). Unlike the majority of individuals in the study, whose samples from different facial regions clustered together, this individual's T-zone was associated with cluster 7 – distinct from the cheek and chin samples, which were in clusters 5 and 4, respectively.
The T-zone in Participant A’s case exhibited significantly higher microbial DNA concentrations, dominated by the Gram-negative bacterium Klebsiella, along with a diverse array of other bacteria, including anaerobes typically involved in polymicrobial infections. This divergent clustering is attributed to folliculitis in the T-zone, highlighting the connection between local skin conditions and the microbiome, and the importance of site-specific sampling in understanding the spatial heterogeneity of the skin microbiome.
Results: Seborrheic Dermatitis and Phage Treatment Case Study
Phages are common on every individual’s skin, but each person hosts a unique yet incomplete collection of specific phages. This means that while certain presumably beneficial phages might be present on one person’s skin, they may be absent on another person’s skin. Furthermore, introducing specific phages topically could induce notable changes in the skin's microbial environment.
In Participant B, described above, who regularly applied the Staphylococcus-specific phage serum for approximately one year, the visible signs of seborrheic dermatitis significantly improved. In addition, the strain-level diversity of Staphylococcus bacteria on his forehead shifted dramatically (see Figure 4, below).
More specifically, the overall composition of Staphylococcus species remained unchanged but a noticeable increase was observed in both the diversity and quantity of Staphylococcus strains; particularly S. epidermidis. What’s more, the Staphylococcus-specific phage, which was undetectable in the initial June 2023 sample, was present in the June 2024 sample, indicating successful integration into the microbiome.
An important note, seborrheic dermatitis is typically associated with Malassezia species but the causal link has not been demonstrated with Koch’s postulates. Some peer-reviewed publications have shown that S. aureus is significantly more common on seborrheic dermatitis lesions than healthy skin.13 Others have speculated that Staphylococcus species compromise the skin barrier in cases of seborrheic dermatitis, either contributing to or primarily causing symptoms.14 Still other studies have found that in the Chinese population, the occurrence of Malessezia does not differ between people with and without seborrheic dermatitis.15
While this case study offers anecdotal evidence and is an outlier without replication, it may also represent a minority of cases wherein Malassezia is not the primary cause. Additional data is required.
In the meantime, in Participant B, Malassezia was not detected despite previously being routinely detected by the described test, and a strain-level shift was observed in the Staphylococcus population after applying a purified, highly targeted anti-Staphylococcus phage.
Discussion: Understanding Site-specific Microbe Variations
The findings of this study underscore the importance of understanding site-specific variations in the skin microbiome for advancing dermatological health and diagnostics. Through quantitative WGS, significant differences were discernible in microbial diversity and composition across distinct facial regions: the T-zone, cheeks and chin.
These differences not only highlight the unique microbial communities associated with each skin site, but also emphasize the need for a more nuanced approach to skin diagnostics and treatment. One striking example from the present study is the case of Participant A, whose T-zone microbiome deviated significantly from typical patterns due to folliculitis, driven by an overgrowth of Klebsiella.
This case challenges conventional diagnostic criteria, as the individual was diagnosed with acne – a condition often attributed to the overgrowth of Cutibacterium acnes.16, 17 However, the presence of Klebsiella suggests the condition was more accurately folliculitis, masquerading as acne.
Furthermore, a particularly unexpected outcome of this study was observed in a Participant B with persistent seborrheic dermatitis, treated using Staphylococcus-specific phage. As noted, although seborrheic dermatitis is typically associated with Malassezia species, the treatment was tailored to reduce S. aureus due to the overgrowth of Staphylococcus species on his face.11, 16
After nearly one year of regular application, not only did the visible signs of seborrheic dermatitis significantly improve, but the strain-level diversity of Staphylococcus on his forehead dramatically shifted. This increase in Staphylococcus diversity, especially of S. epidermidis, was only detected by WGS DNA sequencing, underscoring the importance of capturing fine-grained microbial changes.
Exploring Phage Therapy
One of the most promising avenues explored in this study is the application of phage therapy to target and manage the skin microbiome. Phages, or bacteriophages, are nano-microbes – i.e., viruses that specifically infect and lyse bacteria. Unlike broad-spectrum antibiotics, which can disrupt the microbiome, cause new infections or select for the growth of antibiotic-resistant strains, phages offer a highly specific method of targeting pathogenic bacteria while preserving beneficial microbes.19 This precision aligns with the findings of our study.
It is important to acknowledge that changing the microbiome long-term with prebiotics, probiotics or postbiotics can be difficult; engraftment is particularly challenging. However, phages and pre/pro/postbiotics modulate the microbiome through distinct mechanisms.
Phages selectively eliminate specific bacterial strains via targeted infection and lysis, reducing their abundance. In contrast, prebiotics fuel beneficial bacteria, probiotics introduce live strains, and postbiotics deliver microbial metabolites, all working to enhance microbiome balance. While phages operate through a strictly subtractive mechanism, removing bacteria, and pre/pro-biotics enhance microbial growth (additive), post-biotics can be either additive or subtractive due to the wide range of metabolites and modes of action.
The implications of phage therapy in dermatology are profound. Custom phage serums could target specific bacteria implicated in skin conditions such as acne, eczema or rosacea. Still, the field of skin microbiome science is early, and several challenges must be addressed before the full potential of microbiome-based therapies can be realized.
One significant limitation is the lack of standardized methodologies and reference databases for skin microbiome studies. The heterogeneity of existing data, coupled with the variability in sampling techniques and analytical methods, complicates the interpretation of results and hinders the development of universal diagnostic and therapeutic guidelines.
Furthermore, the dynamic nature of the skin microbiome, influenced by a myriad of factors such as diet, lifestyle and environmental exposures, adds another layer of complexity to understanding its role in health and disease.20
Notably, the U.S. Food and Drug Administration has not yet approved phage therapy for clinical use to treat atopic dermatitis or any other disease, although three phage trials, sponsored by Armata Pharmaceuticals,21 Locus Biosciences22 and BiomX,23 have progressed to late Phase II with encouraging results to treat cystic fibrosis and UTIs. One of the major hurdles with phage trials of the past is that they have lacked the sequencing and phage manufacturing technologies available today to identify the precise bacterial target, create an appropriate phage or phage “cocktail,” and produce the phage at high enough concentration. The authors' company continues to develop these areas.
The current study, while providing valuable insights, also highlights the need for more comprehensive research to establish a clearer picture of the skin microbiome's structure and function. Future studies should control for variability, with carefully planned sample collection and the introduction of reliable quantification methods to gain a realistic view of how the skin microbiome changes over time in response to host, environment and intervention. Longitudinal studies, in particular, are important to understanding how the skin microbiome changes over time and in response to various interventions, including phage therapy.
Conclusions
This study provides valuable insights into the site-specific variations of the facial skin microbiome and their implications for dermatological health. The integration of WGS into skin diagnostics presents a powerful tool for understanding these variations, and the potential application of phage therapy offers a promising, targeted approach to managing skin health.
As research in this area continues to evolve, phage therapy could become an integral part of personalized skin care, providing effective solutions for a wide range of conditions while preserving the delicate balance of the skin microbiome. Personalized interventions, tailored to an individual's unique microbial composition, will not only enhance treatment efficacy, but also pave the way for more precise and effective management of skin health.
Footnotes
a Illumina NovaSeq 6000
b MD-03 Phage Protocol and
c Orion Active Phage Serum are products of Parallel Health.x
References
1. Harris-Tryon, T.A. and Grice, E.A. (2022, May 26). Microbiota and maintenance of skin barrier function. Science. Available at https://doi.org/10.1126/science.abo0693
2. Grice, E.A. and Segre, J.A. (2011). The skin microbiome. Nature Reviews. Microbiology, 9(4), 244-253.
3. Meisel, J.S., Hannigan, G.D., ... Grice, E.A., et al. (2016). Skin microbiome surveys are strongly influenced by experimental design. The Journal of Investigative Dermatology, 136(5), 947-956.
4. Wei, Q., Li, Z., Gu, Z., Liu, X., Krutmann, J., Wang, J. and Xia, J. (2022). Shotgun metagenomic sequencing reveals skin microbial variability from different facial sites. Frontiers in Microbiology, 13, 933189.
5. Chen, Y., Knight, R. and Gallo, R.L. (2023). Evolving approaches to profiling the microbiome in skin disease. Frontiers in Immunology, 14, 1151527.
6. Sachdeva, C., Satyamoorthy, K. and Murali, T.S. (2022). Microbial interplay in skin and chronic wounds. Current Clinical Microbiology Reports, 9(3), 21-31.
7. Baron, S.A., Diene, S.M. and Rolain, J.-M. (2018, Dec.) Human microbiomes and antibiotic resistance. Human Microbiome Journal. Available at https://www.sciencedirect.com/science/article/pii/S2452231718300058
8. Natarelli, N., Gahoonia, N. and Sivamani, R.K. (2023). Bacteriophages and the microbiome in dermatology: The role of the phageome and a potential therapeutic strategy. Int J Mol Sci. Available at https://pubmed.ncbi.nlm.nih.gov/36769020/
9. Clum, A., Huntemann, M., ... Ivanova, N.N., et al. (2021). DOE JGI Metagenome Workflow. mSystems. Available at https://doi.org/10.1128/mSystems.00804-20
10. Pierce, N.T., Irber, L., Reiter, T., Brooks, P. and Brown, C.T. (2019). Large-scale sequence comparisons with sourmash. F1000Research. Available at https://pubmed.ncbi.nlm.nih.gov/31508216/
11. Li, J., Feng, ... Shi, D., et al. (2022). Presence of Malassezia hyphae is correlated with pathogenesis of seborrheic dermatitis. Microbiology Spectrum. Available at https://doi.org/10.1128/spectrum.01169-21
12. Mukherjee, S., Mitra, R., Maitra, A., Gupta, S., Kumaran, S., Chakrabortty, A. and Majumder, P.P. (2016). Sebum and hydration levels in specific regions of human face significantly predict the nature and diversity of facial skin microbiome. Scientific Reports, 6(1), 1-11.
13. Tamer et al. 2018 - xxxxx waiting on reference
14. An et al. 2017 - xxxxx waiting on reference
15. Lian et al. 2014 - xxxx waiting on reference
16. Cavallo, I., Sivori, F., Di Domenico, E.G., et al. (2022). Skin dysbiosis and Cutibacterium acnes biofilm in inflammatory acne lesions of adolescents. Scientific Reports, 12(1), 1-16.
17. Giuliano, C., Patel, C.R. and Kale-Pradhan, P.B. (2019). A guide to bacterial culture identification and results interpretation. Pharmacy and Therapeutics, 44(4) 192.
18. Li, H. (2013). Aligning sequence reads, clone sequences and assembly contigs with BWA-MEM. Cornell University. Available at http://arxiv.org/abs/1303.3997
19. Pirnay, J.-P. and Kutter, E. (2021). Bacteriophages: It’s a medicine, Jim, but not as we know it. The Lancet Infectious Diseases, 21(3) 309-311.
20. Dimitriu, P.A., Iker, B., Malik, K., Leung, H., Mohn, W.W. and Hillebrand, G.G. (2019). New insights into the intrinsic and extrinsic factors that shape the human skin microbiome. mBio. Available at https://doi.org/10.1128/mbio.00839-19
21. Armata Pharmaceuticals. (2024, Jul 12). Armata Pharmaceuticals announces positive topline data from Phase 1b/2a SWARM-P.a. clinical trial of inhaled AP-PA02 in patients with cystic fibrosis. Available at https://www.sec.gov/Archives/edgar/data/921114/000110465923028700/tm238594d1_ex99-1.htm
22. Locus Biosciences. (2024, Aug 12). Locus Biosciences announces positive results from Part 1 of ELIMINATE Phase 2 Trial of the engineered bacteriophage therapy LBP-EC01 published in The Lancet Infectious Diseases. Available at https://tinyurl.com/2mmmzd4h
23. BiomX. (2023, Nov 29). BiomX announces positive topline results from Part 2 of the Phase 1b/2a trial evaluating BX004 for treatment of chronic pulmonary infections in patients with cystic fibrosis. Available at https://ir.biomx.com/news-events/press-releases/detail/99/biomx-announces-positive-topline-results-from-part-2-of-the