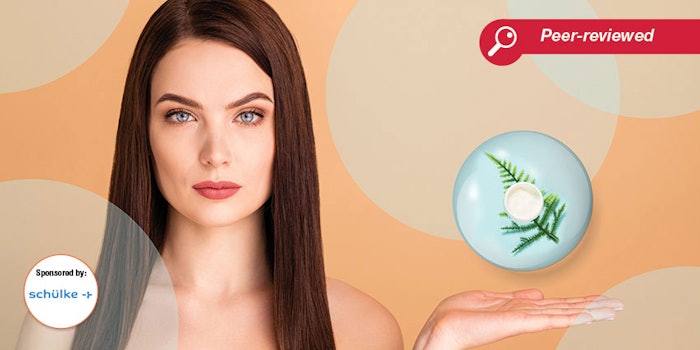
Read this and other articles similar to this in the September 2020 digital edition.
Preservatives protect products from contamination by microorganisms during the product development stage and throughout the product’s lifecycle. They must be present in sufficient quantities to prevent microorganism survival, adaptation and growth in products, which can cause product deterioration and, if used by consumers, cause health issues such as eye infections.1 On the other hand, although preservatives maintain the microbiological quality of products, they can also adversely affect human skin, for example, by causing allergic reactions.2 Since excessive preservatives may pose safety issues, most are regulated in their types, quantities and applications.3-5
See related sidebar: [sponsored] Give Your Products a Boost with schülke
Recently, the number of available preservative options has narrowed, especially for cosmetic products. One preservative that is available is phenoxyethanol (PE), which has been widely and increasingly used as a preservative in cosmetics in recent years.6 PE has broad-spectrum antimicrobial activity and is effective against various Gram-negative and Gram-positive bacteria.7 The future use of PE as a cosmetic preservative requires its optimization, so that it is applied in minimal quantities and with maximal efficacy. One approach to minimize PE quantity is to use preservative boosters such as fatty acids, ethylhexylglycerin, glycols, phenolic antioxidants and chelating agents, which enhance the activity of PE and other preservatives.8 Notably, chelators are used in almost every personal care formulation type to improve product stability and boost preservative activity.9
Product formulations also contain various other components such as oils, polymers, surfactants and plant extracts, and these ingredients can affect preservative efficacy as well. Some directly inhibit preservative efficacy while others may stimulate the growth of microorganisms.10 For example, utmost care should be exercised with high quantities of plant extracts. This is because some extracts exhibit antimicrobial activity while others decrease preservative efficacy.11 Typically, polyphenols and tannins contained in plant extracts have antimicrobial activities.12 On the other hand, saccharides, polypeptides, amino acids and minerals contained in plant extracts may negatively affect preservative efficacy because they act as nutrients for microorganisms, permitting their adaptation and proliferation even in the presence of preservatives. Therefore, to design robust and reliable preservative systems for products containing plant extracts, a detailed understanding of how such extracts influence preservative efficacy and affect microbial adaptation is crucial.
Here, the authors demonstrate the beneficial effects of the combination of PE and ethylenediaminetetraacetic acid (EDTA), a commonly used metal chelator, in skin lotion formulations. As a model case, the booster effects of the latter were investigated in lotions containing high quantities of a proprietary plant extract blend. In addition to determining preservative efficacy, the authors explore how this combination affected microbial adaptation.
Materials and Methods
Bacterial cultures: To evaluate the influence of the aforementioned ingredients under realistic conditions and to design a robust preservative system, environmentally isolated Staphylococcus sp. KMC153 was procured. The bacteria were stored in 10% glycerol at -80°C before use. An aliquot of frozen stock was thawed at room temperature, streaked on Soybean-Casein Digest (SCD) agar, and cultured at 32.5°C for 48 hr. For all experiments, individual aliquots were used once from frozen stock and discarded.
Formulations: General lotion formulations with or without a proprietary plant extract blend were tested for preservative efficacy (see Tables 1 and 2). PE was used at several concentrations and pH values were adjusted to 7.0. A mixture of several plant extracts previously reported to decrease preservative efficacy11 was used as a model extract. EDTA disodium salt was used as a preservative booster.
Preservative efficacy test: Bacterial colonies were collected from the SCD agar and suspended in saline to an optical density at 600 nm (OD600) of 0.2. Following, 50 μL of the resulting suspension was mixed with 5 mL of each model lotion in a glass tube. After 1, 3, 7, 10 and 14 days of incubation at 32.5°C, 100 μL of the test solutions from each tube was drawn, mixed with 900 μL of lecithin-polysorbate 80, diluted and spread onto SCD agar. Colony-forming units (CFU) were counted after a 48-hr incubation at 32.5°C.
Microbial adaptation test: Microbial adaptation levels were determined by comparing the minimum inhibitory concentrations (MICs) of PE before and after culturing in the model lotions. To evaluate the effects of EDTA on microbial adaptation, MICs of cultures grown in lotions with and without EDTA were compared. An individual colony was picked from SCD agar, inoculated in SCD broth and cultured at 37°C with shaking for 16 hr. The cells were centrifuged to a pellet and washed by saline. The pellet was resuspended in saline to an OD600 value of 20 (see process depiction in Figure 1). Following this, 7 μL of the resulting suspension was inoculated into 700 μL of each model lotion and cultured at 37°C for 48 hr.
After culturing in each model lotion, MICs were determined by a slightly modified broth dilution method.13 Briefly, serially diluted PE solutions were prepared in a 96-well microtiter plate using SCD broth. To determine MICs before and after culturing in model lotions, 10 series of PE solutions ranging from 0.696% to 1.02% with a 36 ppm difference between each concentration were prepared. The cells cultured in lotions were adjusted to OD600 value of 0.1 with saline, and 2 μL of the prepared suspension was inoculated into 200 μL of each PE solution. The MICs were determined as the lowest concentration of PE that prevented the visible growth of cells at 37°C for 48 hr.
Statistical analysis: The statistical significance of differences between multiple groups was assessed using the one-way analysis of variance and post-hoc Bonferroni correctiona.
Results: Plant Extracts on Preservative Efficacy
First, the effects of the plant extracts on preservative efficacy in model lotions without preservative boosters were evaluated (Table 1). PE is known to be less effective against Gram-positive bacteria than against Gram-negative bacteria.7, 14, 15 For this reason, a strain of Staphylococcus—Gram-positive bacteria that colonize human skin and a known contaminant of cosmetics—was utilized.16 Here, an expected negative relationship was observed between preservative efficacy and plant extract levels (see Figure 2).
In formulations without plant extracts, the number of surviving cells began to decrease immediately and reached levels below the detection limit within seven days. However, a transient increase in the number of surviving cells was followed by a gradual decrease when plant extracts were present. The number of surviving cells in the lotion with 4.0% plant extracts after a 14-day incubation was almost the same as the initial cell count.
Results: EDTA as a Booster
As noted, to investigate the preservative-boosting effects of EDTA, preservative efficacy with or without this ingredient was measured in eight model lotions (see Tables 1 and 2); a lotion containing 0.4% PE with or without 4.0% plant extracts was used as the control. The boosting effects of 0.05% EDTA were compared with the effects of increasing PE concentrations from 0.4% to 0.5%.
Without plant extracts, preservative efficacy was sufficient in all cases but was the highest in the EDTA-containing lotion with the lowest concentration of PE (see Figure 3). In the presence of plant extracts, preservative efficacy was insufficient even with 0.5% PE.
Only the EDTA-containing formula exhibited high boosting effects (see Figure 4). Furthermore, in the formulas with PE but without EDTA, and there was no significant reduction in the number of surviving cells even after 14 days of incubation. The transient increase in the number of surviving cells at the initial phase also was reduced by EDTA. In addition, supplementation with EDTA resulted in faster bactericidal action (Figure 4).
Results: EDTA and Microbial Adaptation
Prior to the adaptation tests, the initial MIC of PE was measured before culturing cells in the lotions. The initial MIC of PE measured with the isolated strain was 0.85 ± 0.02% (see Figure 5, left), which was higher than the MIC measured with Staphylococcus aureus ATCC6538, the standard strain for preservative efficacy testing under ISO 11930 (data not shown). After culturing in the lotion, without EDTA, MICs were higher than the initial value, indicating that cells acquired resistance to PE during culturing (see Figure 5, center).17 However, MICs did not change after culturing in the EDTA-containing lotion (see Figure 5, right).
Discussion
The present work demonstrates that a combination of PE and EDTA has a robust preservative effect in lotions containing high quantities of nutritional plant extracts, where bacteria cells tend to proliferate. Supplementation with EDTA significantly enhanced preservative efficacy, whereas increasing PE concentration had little effect, especially in the presence of plant extracts.
EDTA promoted a bactericidal effect regardless of plant extract presence. Moreover, the temporary increase in the number of surviving cells, which occurred only in the presence of plant extracts, was reduced by EDTA, suggesting it not only enhanced bactericidal activity, but also inhibited cell proliferation. By chelating Ca2+ and Mg2+ ions that stabilize the lipopolysaccharide layer of Gram-negative bacteria and the peptidoglycan layer of Gram-positive bacteria, EDTA compromises bacterial cell wall and membrane integrity, thereby making cells more sensitive to antimicrobial agents.18, 19 In addition, EDTA sequesters metal ions necessary for microbial metabolism and growth.19, 20 Thus, EDTA has apparently enhanced the bactericidal activity of PE and exhibited its own bacteriostatic activity.
Another interesting finding in this study was the impact of EDTA on microbial adaptation. Microorganisms adapt to various environmental stresses, such as heat, acidic conditions,21 and antimicrobial compounds, including preservatives,22, 23 acquiring a resistance to them. Adapted microorganisms can survive and even grow in products that contain enough preservatives for non-adapted microorganisms. Therefore, it is desirable to design preservative systems that prevent microbial adaptation.
Pseudomonas aeruginosa exhibits adaptive resistance to PE after repetitive exposure to sublethal PE levels.24 Here, we found that adaptation to PE was induced in Staphylococcus sp. when the preservative efficacy of PE was reduced by plant extracts. Thus, Gram-positive bacteria could acquire resistance to PE if it is not used appropriately. MIC did not increase in the EDTA-containing lotion, indicating that EDTA inhibited microbial adaptation to PE.
Microbial adaptation is greatly accelerated when sublethal antimicrobial compounds are added to actively growing cell cultures.25 Products containing plant extracts that act as nutrients for microorganisms facilitate growth and the adaptation of cells to preservatives. Here, EDTA likely prevented cell proliferation by chelating the metal ions needed for growth, consequently inhibiting adaptation to PE.
The present findings demonstrate how boosters can maximize preservative efficacy while minimizing the quantity of preservative required. In fact, supplementation with EDTA was more effective than increasing the PE concentration for both improving preservative efficacy and inhibiting microbial adaptation. Further studies are warranted to understand the mechanisms responsible for boosting activities by EDTA. Preservative efficacy and booster effects clearly will depend on the formulation design and the targeted microorganism. As such, in the future, the applicability of EDTA to various types of formulations will be evaluated, other effective boosters and combinations will be explored, and the mechanisms underlying their actions will be clarified.
Conclusion
The present work demonstrates that plant extracts diminish the preservative efficacy of PE, whereas EDTA boosts PE efficacy and inhibits microbial adaptation. A combination of PE and EDTA, which has both bactericidal and bacteriostatic effects, is therefore proposed as a reliable and robust preservative system, particularly for products containing ingredients that may be used as nutrients by microorganisms.
References
- Reid, F.R. and Wood, T.O. (1979). Pseudomonas corneal ulcer: The causative role of contaminated eye cosmetics. Arch Ophthalmol 97(9) 1640-1641.
- Lundov, M.D., Moesby, L., Zachariae, C. and Johansen, J.D. (2009). Contamination versus preservation of cosmetics: A review on legislation, usage, infections and contact allergy. Contact Dermatitis 60(2) 70-78.
- EU Open Data Portal (accessed 2020, Jul 8). Cosmetic Regulation 1223/2009 Annex V in Europe. Available at: https://data.europa.eu/euodp/en/data/dataset/cosmetic-ingredient-database-2-list-of-substances-prohibited-in-cosmetic-products
- CIR (accessed 2020, Jul 8). A positive Cosmetic Ingredient Review (CIR) list in the United States. Available at: https://www.cir-safety.org/ingredients
- Japanese Ministry of Health and Welfare (accessed 2020, Jul 8). A positive list for preservatives in standards for cosmetics in Japan. Available at: https://www.mhlw.go.jp/file/06-Seisakujouhou-11120000-Iyakushokuhinkyoku/0000032704.pdf
- Schmidt, S., Sedlewicz, L. and Chirico, I. (2019, Sep 19). Preserve and protect: Shielding the sunscreen umbrella. Cosm & Toil. Available at: https://www.cosmeticsandtoiletries.com/formulating/category/suncare/Preserve-and-Protect-Shielding-the-Sunscreen-Umbrella-558845941.html
- Puschmann, J., Herbig, M.E., and Müller-Goymann, C.C. (2018). Correlation of antimicrobial effects of phenoxyethanol with its free concentration in the water phase of o/w-emulsion gels. Eur J Pharm Biopharm 131 152-161.
- Herman, A. (2019). Antimicrobial ingredients as preservative booster and components of self-preserving cosmetic products. Curr Microbiol 76(6) 744-754.
- Abrutyn, E.S. (2013, Jul 17). Deciphering chelating agent formulas. Cosm & Toil. Available at https://www.cosmeticsandtoiletries.com/formulating/function/aids/premium-deciphering-chelating-agent-formulas-215885521.html
- Araki, H., Hamada, S., Toshima, Y., Kawazoe, Y., Otani, M., Suzuki, R., Usukura, J. and Okawa, M. (2018, Feb 26). An offensive defense: Minimizing preservatives, maximizing protection and resources. Cosm & Toil 133(3) 65-78. Available at https://www.cosmeticsandtoiletries.com/formulating/function/preservatives/An-Offensive-Defense-Minimizing-Preservatives-Maximizing-Protection-and-Resources-475204783.html
- Kubota, H., Endo, K. and Tanahashi, M. (2005, Jul 28). JP Pat 2005-200395A. Blended material of agent for external use. Assigned to Kao Corp.
- Cowan, M.M. (1999). Plant products as antimicrobial agents. Clin Microbiol Rev 12(4) 564-582.
- Wiegand, I., Hilpert, K. and Hancock, R.E. (2008). Agar and broth dilution methods to determine the minimal inhibitory concentration (MIC) of antimicrobial substances. Nat Protoc 3(2) 163-175.
- Roden, K. (2010). Preservatives in personal care products. Microbiol Aust 31(4) 195-197.
- Meyer, B.K., Ni, A., Hu, B. and Shi, L. (2007). Antimicrobial preservative use in parenteral products: Past and present. J Pharm Sci 96(12) 3155-3167.
- Campana, R., Scesa, C., Patrone, V., Vittoria, E. and Baffone, W. (2006). Microbiological study of cosmetic products during their use by consumers: Health risk and efficacy of preservative systems. Lett Appl Microbiol 43(3) 301-306.
- Brauner, A., Fridman, O., Gefen, O. and Balaban, N.Q. (2016). Distinguishing between resistance, tolerance and persistence to antibiotic treatment. Nature Reviews Microbiology 14(5), 320-330.
- Hansen, L.T., Austin, J.W., and Gill, T.A. (2001). Antibacterial effect of protamine in combination with EDTA and refrigeration. Int J Food Microbiol 66(3) 149-161.
- Varvaresou, A., Papageorgiou, S., Tsirivas, E., Protopapa, E., Kintziou, H., Kefala, V. and Demetzos, C. (2009). Self-preserving cosmetics. Int J Cosmet Sci 31(3) 163-175.
- Reidmiller, J.S., Smith, W.L., Sawyer, M.M., Osburn, B.I., Stott, J.L., and Cullor, J.S. (2006). Antimicrobial properties of the chelating agent EDTA on Streptococcal bovine mastitis isolates. J Food Prot 69(6) 1460-1462.
- Cebrián, G., Sagarzazu, N., Pagán, R., Condón, S. and Mañas, P. (2010). Development of stress resistance in Staphylococcus aureus after exposure to sublethal environmental conditions. Int J Food Microbiol 140(1) 26-33.
- McBain, A.J., Ledder, R.G., Moore, L.E., Catrenich, C.E. and Gilbert, P. (2004). Effects of quaternary-ammonium-based formulations on bacterial community dynamics and antimicrobial susceptibility. Appl Environ Microbiol 70(6) 3449-3456.
- Rushton, L., Sass, A., Baldwin, A., Dowson, C.G., Donoghue, D. and Mahenthiralingam, E. (2013). Key role for efflux in the preservative susceptibility and adaptive resistance of Burkholderia cepacia complex bacteria. Antimicrob Agents Chemother 57(7) 2972-2980.
- Adbel Malek, S. and Badran, Y.R. (2010). Pseudomonas aeruginosa PAO1 adapted to 2-phenoxyethanol shows cross-resistance to dissimilar biocides and increased susceptibility to antibiotics. Folia Microbiologica (Praha) 55(6) 588-592.
- Russell, A.D. (2004). Bacterial adaptation and resistance to antiseptics, disinfectants and preservatives is not a new phenomenon. J Hosp Infect 57(2) 97-104.