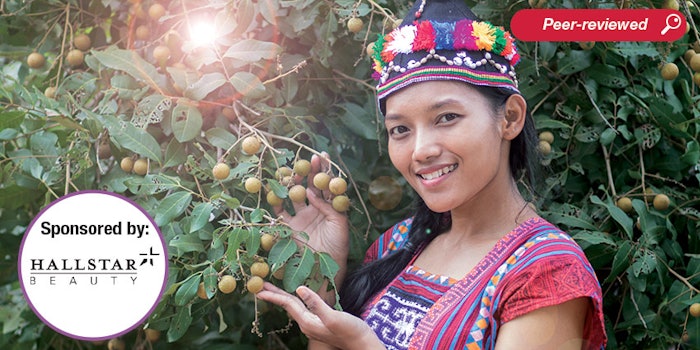
Read the full article in the July/August 2021 digital edition. . .
Similarly to plants, skin is exposed to light for most of the day and is at risk for photo-oxidative stress. While plants are uncontrollably exposed to most if not all wavelengths of solar radiation, the exposure of skin is instead based on an individual’s activities (see Figure 1).
Sidebar: Turmeric-powder Protection
Sunlight has a broad spectrum of wavelengths that can be roughly divided into visible light (including blue light, the portion closer to UV); UV light; and long wavelength infrared radiation. The longer the wavelength, the deeper it can penetrate skin. Infrared and visible light can reach the lower layers,1 whereas short wavelengths such as UV are absorbed mainly by the superficial skin layers, which is known to induce inflammation.2
Sun protection products often include synthetic solar filter molecules to protect skin. Although highly effective, the sustainability of these filters, like other cosmetic components, has been challenged in part due to the potential risk they pose to the environment.3-5 This is because their biodegradability is hindered by their complex aromatic structure and size.3-5
As an alternative, plants could offer new strategies for sun protection; in fact, review articles in recent years have focused on natural UV filters.6-8 Previous work9 envisioned the similarities between the life of plants and skin. The present article looks specifically at the effects of light in both skin and plants. It also explores how the evolutionary mechanisms in plants to fight light-induced stress—could translate to sun protection for skin.
Effects of Light in Skin
Both human skin and plants have evolved mechanisms to respond to the consequences of light exposure. For example, studies have shown that keratinocytes can sense light and that they have their own tight circadian rhythm.10 These mechanisms act by absorbing damaging radiation, scattering it and/or reflecting it, while also fighting the formation of free radicals that can interfere with cellular machineries.
As is well-known, skin’s long-term exposure to sunlight results in a leathery and lax appearance, with significant wrinkles, visible red capillaries and melanized spots.11 Photoaging is also characterized by the accumulation of aggregated elastin fibers in the upper dermis that results in its lack of elasticity and resilience.11 On a cellular level, exposure to light and high energy radiation such as UV leads to the production of reactive oxygen species (ROS) that can react with proteins and enzymes.12
Compared with past decades, the skin is also now more exposed to artificial light and light emitted by electronic devices. The effects of these exposures have recently been investigated especially in the context of the pandemic and rise in the use of screens for communication and smart working solutions.13
The light emitted by smartphones and laptops is predominantly distributed between 440 nm and 460 nm14 and has not only has been reported to affect sleeping quality,15 but also the skin; although some debate surrounds this.16 Still, some studies have shown artificial light increases the production of reactive oxygen species.17 Others indicate that blue light at 410 nm increases inflammatory mediators, causing DNA damage while altering the circadian rhythm of cells.18
There also are microbial considerations in skin. In resident skin bacteria, short wavelength visible light such as that emitted by smartphones and tablets has been shown to promote the proliferation of Staphylococcus aureus,19, 20 although this light has been successfully used to fight acne-causing bacteria such as Propionibacterium acnes with local treatment and at a different intensity.20
Effects of Light in Plants— and Opportunities
Plants also sense blue light, which is an important regulator of phototropism, chloroplast movements, stomata opening and leaf thickness.21, 22 Blue light ensures the efficiency of the whole photosynthetic process as well.23 Studies in plants such as Dimocarpus longan (longan) have shown how they respond to blue light by synthesizing flavonoids, epicatechin and rutin.24 To counteract the aggression of solar radiation, plants also have evolved a plethora of secondary metabolites that, as noted, could find roles in cosmetic preparations to protect skin (see Table 1).
For example, in tea plants, exposure to intense blue light inhibited the expression of biosynthetic pathways of lipids while promoting the synthesis of some flavonoids.31 This response, however, is plant-specific.32 Similarly, blue light exposure has been reported to enhance the concentration of secondary metabolites such as flavonoids and anthocyanins in fruits.33, 34 Even common plants such as eggplant30 or guava fruit27 have been shown to absorb light.
The flavonoids produced by plants as secondary metabolites are characterized by their polyphenolic structures, which intrinsically can absorb UV radiation. They have two absorption maximums in the ranges 240-285 nm and 300-400 nm,35 which help to prevent both damages to DNA and protein molecules, and the generation of reactive oxygen species.36 These molecules shield the plant from excessive solar radiation, acting similarly to the filters used in sunscreens. Also, compared with synthetic molecules that, as noted, can bioaccumulate in the environment, flavonoids are easily transformed by the enzymes and microbes available in nature.37
Besides light absorption, the antioxidant molecules in plants can help to support the natural antioxidant mechanism in skin by preventing the accumulation of radicals in situ.38 In fact, in plants, most secondary metabolites whose synthesis are promoted by light exposure also have significant antioxidant activity. These include not only flavonoids but also, e.g., hydroxytyrosol from olives, which has been shown to protect protein residues from undergoing modification via the formation of abnormal L-isoaspartyl residues.39 Furthermore, flavonoids can be added to formulations to stabilize UV filters.38
Whereas many studies focus on plant extracts and their flavonoid-rich compositions, plant-derived structural molecules and isolated compounds also are promising ingredients with functionality similar to sunscreens or sunscreen boosters (see Table 2). For example, small molecules such as rutin40 and hydroxytyrosol,42 a strong antioxidant, have shown efficacy as sunscreens or in support of other sunscreens. From a different approach, lignin is a high molecular weight aromatic molecule and by-product of the paper industry that offers a potential sustainable alternative to chemical light filters.41 A polymer such as nanocellulose also has been used to immobilize synthetic light filter molecules, allowing for their reduced levels to achieve an equivalent SPF.43
Sustainability and Technical Considerations
As stated, using plant-derived ingredients in place of synthetic UV filters could circumvent the problem of bioaccumulation. Most of the plants explored for their light protection potential, however, are known from traditional medicine. As such, attention must be given to ensure their sustainable harvest while promoting a change from wild picking, to planned cultivation, to in vitro production.44, 45 As an alternative to intensive cultivation, plants also could be grown in a diffused local approach, thus serving as a tool for social progress and empowering local economies.46
The potential incorporation of these ingredients into formulations, however, carries with it some technical considerations. One aspect is the reported SPF values in the literature. These are often measured using methodologies that are not directly comparable, e.g., in vitro, in vivo or spectrophotometrically. Moreover, the SPF values are often calculated for extracts alone, not incorporated into formulations, so it is key to assess their efficacy under appropriate conditions. For instance, a value as high as SPF 20 was reported in one case for a Rosa kordesii extract, whereas in a formulation, it achieved only an SPF of 3.47
Another is the use of unsuitable solvents, such as methanol, which limits the straightforward adoption of the natural ingredient into the formula. And complex preparations of plant extracts can undergo undesired side reactions leading to the polymerization, degradation, precipitation, etc., of the compound of interest.48, 49 The production and storage of polyphenolic-rich plant extracts may therefore require adapted processes to ensure the maximum presence of bioactive compounds and preserve their functionality.50
Furthermore, not affecting performance but appearance is the intrinsic color of many plant extracts and their light-absorbing or antioxidant molecules at an effective concentration, which might limit their use in a final product. However, their addition to confer a low SPF, for example in day cream, should be feasible; their higher potential may be found in the role of boosting synthetic filter molecules to lower the required concentrations for efficacy.
In one case, with guava fruit extract, a reduction of more than 70% in the concentration of total synthetic organic filters was possible while still achieving an SPF of 18.51 This is relevant for environmentally conscious development, considering both the production phase and the post-consumer phase of synthetic UV filters. The use of natural alternatives also helps to align with the 17 Sustainable Development Goals set forth by the UN but importantly, also can impact the cost of the final product especially in the short-term when production is not yet adequately planned.
Summary
Plants can offer a helping hand to cosmetic developers looking for natural and sustainable ingredients not only for their health-promoting activities, but also in the field of sun protection. Exposure to sunlight triggers the formation of reactive species in both plant and human skin cells, and plants have evolved a wide array of molecules capable of absorbing this solar radiation either directly or to contrast its damaging effects inside cells. These functional molecules have been studied as both plant extracts and as isolated molecules, and many have shown protective effects even when included in cosmetic formulations.
In summary, plant-derived ingredients can impact cosmetic products beyond their envisioned function, albeit with some caveats (see Figure 2). Importantly, they introduce a different supply chain with characteristic connections to their territory usage, environmental impact and population, and thus provide an opportunity to improve the sustainability of the whole sector.
References
- Krutmann, J., et al. (2017). The skin aging exposome. J Derm Sci 85(3) 152-161.
- Hruza, L.L. and Pentland, A.P. (1993). Mechanisms of UV-induced inflammation. J Inves Derm 100 1 suppl S35-S41.
- Sharifan, H., Klein, D. and Morse, A.N. (2016). UV filters are an environmental threat in the Gulf of Mexico: A case study of Texas coastal zones. Oceanologia 58 4 327-335.
- Bom, S., Ribeiro, H.M. and Marto, J. (2020). Sustainability calculator: A tool to assess sustainability in cosmetic Products [abstract]. Sustainability 12(4).
- Bom, S., et al. (2019). A step forward on sustainability in the cosmetics industry: A review. J Cleaner Production 225 270-290.
- Cefali, L.C., et al. (2016). Plant-based active photoprotectants for sunscreens. Intl J Cos Sci 38 4 346-353.
- Radice, M., et al. (2016). Herbal extracts, lichens and biomolecules as natural photo-protection alternatives to synthetic UV filters. A systematic review. Fitoterapia 114.
- Pandika, M. (2018). Looking to nature for new sunscreens. ACS Central Science 4 7 788-790.
- Faccio, G. (2020). Plant complexity and cosmetic innovation. iScience 23 8 101358.
- Matsui, M.S., et al. (2016) Biological rhythms in the skin. Intl J Mol Sci 17 6 801.
- Rittié, L. and Fisher, G.J. (2015). Natural and sun-induced aging of human skin. Cold Spring Harbor Perspectives in Medicine 5 1.
- de Jager, T.L., Cockrell, A.E. and Du Plessis, S.S. (2017). Ultraviolet light-induced generation of reactive oxygen species. Adv Exp Med Biol 996 15-23.
- Jakhar, D., Kaul, S. and Kaur, I. (2020). Increased usage of smartphones during COVID-19: Is that blue light causing skin damage? J Cos Derm 19 10 2466-2467.
- Moyano, D.B., Sola, Y. and González-Lezcano, R.A. (2020). Blue-light levels emitted from portable electronic devices compared to aunlight [abstract]. Energies 13(16).
- Mortazavi, S.A.R., Parhoodeh, S., ... Mortazavi, S.M.J., et al. (2018). Blocking short-wavelength component of the visible light emitted by smartphones' screens improves human sleep quality [abstract]. Available at: https://pubmed.ncbi.nlm.nih.gov/30568927/
- Grabenhofer, R. (2021, May 5). Beiersdorf refutes reports that artificial blue light causes skin damage. Available at: https://www.cosmeticsandtoiletries.com/research/biology/Beiersdorf-Refutes-Reports-that-Artificial-Blue-Light-Causes-Skin-Damage_574355941.html
- Mironava, T., et al. (2012). The effects of UV emission from compact fluorescent light exposure on human dermal fibroblasts and keratinocytes in vitro. Photochem and Photobiol 88 6 1497-1506.
- Dong, K., et al. (2019). Blue light disrupts the circadian rhythm and create damage in skin cells. Int J Cos Sci 41 6 558-562.
- Taheri, M., Darabyan, M., ... Moradi, M., et al. (2017). Exposure to visible light emitted from smartphones and tablets increases the proliferation of Staphylococcus aureus: Can this be linked to acne? [abstract]. Available at: https://pubmed.ncbi.nlm.nih.gov/28580338/
- Dai, T., et al. (2012). Blue light for infectious diseases: Propionibacterium acnes, Helicobacter pylori and beyond? Drug Resistance Updates 15 4 223-236.
- Lin, C. (2002). Blue light receptors and signal transduction. The Plant Cell 14 suppl S207-S225.
- Zheng, L. and Van Labeke, M. (2017). Long-term effects of red- and blue-light emitting diodes on leaf anatomy and photosynthetic efficiency of three ornamental pot plants. Frontiers in Plant Science 8 917.
- Hogewoning, S.W., et al. (2010). Blue light dose–responses of leaf photosynthesis, morphology and chemical composition of Cucumis sativus grown under different combinations of red and blue light. J Exper Botany 61 11 3107-3117.
- Li, H., et al. (2018). Effects of blue light on flavonoid accumulation linked to the expression of miR393, miR394 and miR395 in longan embryogenic calli. PloS One 13 1 e0191444-e0191444.
- Morvan, P., et al. (2019). Effects of blue light on human skin and the protective effect of Artemisia capillaris extract. IFSCC Mag 22 93-99.
- Mejía-Giraldo, J.C., et al. (2016). Photoprotective potential of Baccharis antioquensis (Asteraceae) as natural sunscreen. Photochem and Photobio 92 5 742-752.
- Mota, M.D., et al. (2019). Guava-fruit extract can improve the UV-protection efficiency of synthetic filters in sun cream formulations. J Photochem and Photobio B: Biology 201 111639.
- Costa, S.C.C., et al. (2015). In vitro photoprotective effects of Marcetia taxifolia ethanolic extract and its potential for sunscreen formulations. Revista Brasileira De Farmacognosia 25 4 413-418.
- Seok, J.K., et al. (2016). Scutellaria radix extract as a natural UV protectant for human skin. Phytother Res 30 3 374-379.
- Sharma, T., Tyagi, V. and Bansal, M. (2020). Determination of sun protection factor of vegetable and fruit extracts using UV–visible spectroscopy: A green approach. Sustainable Chem and Pharma 18 100347.
- Wang, P., Chen, S., ... Ye, N., et al. (2020). Exploration of the effects of different blue LED light intensities on flavonoid and lipid metabolism in tea plants via transcriptomics and metabolomics [abstract]. Int J Molec Sci 21(13).
- Taulavuori, K., et al. (2018). Responses of phenolic acid and flavonoid synthesis to blue and blue-violet light depends on plant species. Envt and Exper Botany 150 183-187.
- Zoratti, L., et al. (2014). Light-controlled flavonoid biosynthesis in fruits. Front Plant Sci 5 534.
- D'Angelo, S., et al. (2005). Hydroxytyrosol, a natural antioxidant from olive oil, prevents protein damage induced by long-wave ultraviolet radiation in melanoma cells. Available at: https://pubmed.ncbi.nlm.nih.gov/15749387/
- Markham, K.R. and Mabry, T.J. (1975). Ultraviolet-visible and proton magnetic resonance spectroscopy of flavonoids. Harborne, J.B., Mabry T.J. and Mabry, H. (eds) The Flavonoids. Springer. 45-77.
- Cefali, L.C., et al. (2016). Plant-based active photoprotectants for sunscreens. Intl J Cos Sci 4 346-353.
- Das, S. and Rosazza, J.P.N. (2006). Microbial and enzymatic transformations of flavonoids. J Nat Products 69 3 499-508.
- Lorigo, M. and Cairrao, E. (2019). Antioxidants as stabilizers of UV filters: An example for the UV-B filter octylmethoxycinnamate. Biomed Dermatol 3 1 11.
- Khalid, M., et al. (2019). Role of flavonoids in plant interactions with the environment and against human pathogens—A review. J Integrative Agriculture 18 1 211-230.
- Velasco, M.V. R., et al. (2008). Associação da rutina com p-metoxicinamato de octila e benzofenona-3: Avaliação in vitro da eficácia fotoprotetora por espectrofotometria de refletância. Lat Am J Pharm 27 23-27.
- Qian, Y., Zhong, X., Li, Y. and Qiu, X. (2017). Fabrication of uniform lignin colloidal spheres for developing natural broad-spectrum sunscreens with high sun protection factor [abstract]. Indus Crops and Prod 101 54-60.
- Guo, W., et al. (2010). The protective effects of hydroxytyrosol against UVB-induced DNA damage in HaCaT cells. Phytother Res 24 3 352-359.
- Zhang, Z., et al. (2019). Cinnamate-functionalized cellulose nanocrystals as UV-Shielding nanofillers in sunscreen and transparent polymer films. Adv Sustain Sys 3 4 1800156.
- van Wyk, A.S. and Prinsloo, G. (2018). Medicinal plant harvesting, sustainability and cultivation in South Africa. Biolog Conserv 227 335-342.
- Chen, S., et al. (2016). Conservation and sustainable use of medicinal plants: Problems, progress and prospects.Chinese Med 11 1 37.
- Hufad, A., Pramudia, J. and Hilmi, M. (2017) The empowerment of environmentally friendly women. Available at: https://bit.ly/3yxMY2j
- Maske, P.P., et al. (2013). In vitro determination of sun protection factor and chemical stability of Rosa kordesii extract gel. J Pharm Res 7 6 520-524.
- Lu, Q., Li, L., Xue, S., Yang, D. and Wang, S. (2019). Stability of flavonoid, carotenoid, soluble sugar and vitamin C in ‘cara cara’ juice during storage [abstract]. Foods 8(9)
- Ali, A., et al. (2018), Impact of storage conditions on the stability of predominant phenolic constituents and antioxidant activity of dried Piper betle extracts. Molecules 23 2 484.
- Ammendola, M., et al. (2020). Stability and anti-proliferative properties of biologically active compounds extracted from Cistus L. after sterilization treatments. Sci Reports 10 1 6521.
- Mota, M.D., et al. (2019). Guava-fruit extract can improve the UV-protection efficiency of synthetic filters in sun cream formulations. J Photochem and Photobio B: Biology 201 111639.